Design of biomedical gradient porous scaffold via a minimal surface dual-unit continuous transition connection strategy
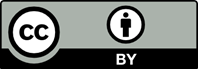
In this work, a series of new gradient porous scaffolds were innovatively designed via a dual-unit continuous transition connection strategy based on the minimal surface structures (primitive [P], diamond [D], and gyroid [G]). The scaffolds were successfully prepared through selective laser melting technology. The results showed that the dual-unit continuous transition connection strategy significantly improved the mechanical properties of the connected scaffolds. The compression strength of the scaffolds was found to be (P-G)>(P-D)>(G-P)>(G-D)>(D-G)>(D-P), with the P-G structure exhibiting a compression strength of 167.7 MPa and an elastic modulus of 3.3 GPa. The mechanical properties of the porous scaffolds were primarily influenced by the outer unit type, the connection condition between different units, the unit size, and the porosity. Scaffolds with the outer P unit demonstrated better mechanical properties due to the higher mechanical strength of the P structure. The connection performance between different units varied, with P and G units forming a good continuous transition connection, while the connection performance between P and D units was the weakest. The dual-unit continuous transition connection strategy offers a promising approach to optimize the connection performance of different units, providing new insights into the design of medical porous scaffolds.
- Moiduddin K, Darwish S, Al-Ahmari A, Elwatidy S, Mohammad A, Ameen W. Structural and mechanical characterization of custom design cranial implant created using additive manufacturing. Electron J Biotechnol. 2017;29:22–31. doi: 10.1016/j.ejbt.2017.06.005
- Gómez S, Vlad MD, López J, Fernández E. Design and properties of 3D scaffolds for bone tissue engineering. Acta Biomater. 2016;42:341–350. doi: 10.1016/j.actbio.2016.06.032
- Henkel J, Woodruff MA, Epari DR, et al. Bone regeneration based on tissue engineering conceptions — A 21st century perspective. Bone Res. 2013;1(1):216–248. doi: 10.4248/BR201303002
- Park S, Park M, Lee B-T. Autologous stromal vascular fraction-loaded hyaluronic acid/gelatin-biphasic calcium phosphate scaffold for bone tissue regeneration. Mater Sci Eng C. 2022;132:112533. doi: 10.1016/j.msec.2021.112533
- Kamal M, Ziyab AH, Bartella A, et al. Volumetric comparison of autogenous bone and tissue-engineered bone replacement materials in alveolar cleft repair: A systematic review and meta-analysis. Br J Oral Maxillofac Surg. 2018;56(6): 453–462. doi: 10.1016/j.bjoms.2018.05.007
- Cui Y-W, Chen L-Y, Chu Y-H, et al. Metastable pitting corrosion behavior and characteristics of passive film of laser powder bed fusion produced Ti–6Al–4V in NaCl solutions with different concentrations. Corros Sci. 2023;215:111017. doi: 10.1016/j.corsci.2023.111017
- Wang L, Xie L, Lv Y, et al. Microstructure evolution and superelastic behavior in Ti-35Nb-2Ta-3Zr alloy processed by friction stir processing. Acta Materialia. 2017;131:499– 510. doi: 10.1016/j.actamat.2017.03.079
- Wang L, Lu W, Qin J, Zhang F, Zhang D. Microstructure and mechanical properties of cold-rolled TiNbTaZr biomedical β titanium alloy. Mater Sci Eng A. 2008;490:421–426. doi: 10.1016/j.msea.2008.03.003
- Guo L, Ataollah Naghavi S, Wang Z, et al. On the design evolution of hip implants: A review. Mater Des. 2022;216:110552. doi: 10.1016/j.matdes.2022.110552
- Barba D, Alabort E, Reed RC. Synthetic bone: Design by additive manufacturing. Acta Biomater. 2019;97:637–656. doi: 10.1016/j.actbio.2019.07.049
- Zadpoor AA. Meta-biomaterials. Biomater Sci. 2019;8(1): 18–38. doi: 10.1039/C9BM01247H
- Xiao R, Feng X, Fan R, et al. 3D printing of titanium-coated gradient composite lattices for lightweight mandibular prosthesis. Composites Part B. 2020;193:108057. doi: 10.1016/j.compositesb.2020.108057
- Liu C, Wang C-Y, Liu H, Wang Z-H, Lin GY. Mechanical properties and biocompatibility of 3D printing Ti6Al4V titanium alloy scaffolds. Chin J Nonferrous Met. 2018;28: 758–765. doi: 10.19476/j.ysxb.1004.0609.2018.04.14
- Song C, Liu L, Deng Z, et al. Research progress on the design and performance of porous titanium alloy bone implants. J Mater Res Technol. 2023;23:2626–2641. doi: 10.1016/j.jmrt.2023.01.155
- Kelly CN, Francovich J, Julmi S, et al. Fatigue behavior of As-built selective laser melted titanium scaffolds with sheet-based gyroid microarchitecture for bone tissue engineering. Acta Biomater. 2019;94:610–626. doi: 10.1016/j.actbio.2019.05.046
- Castro APG, Pires T, Santos JE, Gouveia BP, Fernandes PR Permeability versus design in TPMS scaffolds. Materials. 2019;12(8):1313. doi: 10.3390/ma12081313
- Zhao B, Gain AK, Ding W, Zhang L, Li X, Fu Yucan. A review on metallic porous materials: pore formation, mechanical properties, and their applications. Int J Adv Manuf Technol. 2018;95(5–8):2641–2659. doi: 10.1007/s00170-017-1415-6
- Chen SY, Kuo CN, Su YL, et al. Microstructure and fracture properties of open-cell porous Ti-6Al-4V with high porosity fabricated by electron beam melting. Mater Charact. 2018;138:255–262. doi: 10.1016/j.matchar.2018.02.016
- Li J, Cui X, Hooper GJ, Lim KS, Woodfield TBF. Rational design, bio-functionalization and biological performance of hybrid additive manufactured titanium implants for orthopaedic applications: A review. J Mech Behav Biomed Mater. 2020;105:103671. doi: 10.1016/j.jmbbm.2020.103671
- Yang N, Quan Z, Zhang D, Yanling T. Multi-morphology transition hybridization CAD design of minimal surface porous structures for use in tissue engineering. Comput Aided Des. 2014;56:11–21. doi: 10.1016/j.cad.2014.06.006
- Van Bael S, Kerckhofs G, Pyka MMG, Schrooten J, Kruth JP. Micro-CT-based improvement of geometrical and mechanical controllability of selective laser melted Ti6Al4V porous structures. Mater Sci Eng A. 2011;528(24):7423–7431. doi: 10.1016/j.msea.2011.06.045
- Lei H-Y, Li J-R, Xu Z-J, Wang Q-H. Parametric design of Voronoi-based lattice porous structures. Mater Des. 2020;191:108607. doi: 10.1016/j.matdes.2020.108607
- Wang G, Shen L, Zhao J, et al. Design and compressive behavior of controllable irregular porous scaffolds: Based on voronoi-tessellation and for additive manufacturing. ACS Biomater Sci Eng. 2018;4(2):719–727. doi: 10.1021/acsbiomaterials.7b00916
- McGregor M, Patel S, McLachlin S, Vlasea M. Architectural bone parameters and the relationship to titanium lattice design for powder bed fusion additive manufacturing. Addit Manuf. 2021;47:102273. doi: 10.1016/j.addma.2021.102273
- Giannitelli SM, Accoto D, Trombetta M, Rainer A. Current trends in the design of scaffolds for computer-aided tissue engineering. Acta Biomater. 2014;10(2):580–594. doi: 10.1016/j.actbio.2013.10.024
- Rajagopalan S, Robb RA. Schwarz meets Schwann: Design and fabrication of biomorphic and durataxic tissue engineering scaffolds. Med Image Anal. 2006;10(5):693–712. doi: 10.1016/j.media.2006.06.001
- Feng J, Fu J, Yao X, He Y. Triply periodic minimal surface (TPMS) porous structures: From multi-scale design, precise additive manufacturing to multidisciplinary applications. Int J Extrem Manuf. 2022;4(2):022001. doi: 10.1088/2631-7990/ac5be6
- Feng J, Wei D, Zhang P, et al. Preparation of TiNbTaZrMo high-entropy alloy with tunable Young’s modulus by selective laser melting. J Manuf Process. 2023;85: 160–165. doi: 10.1016/j.jmapro.2022.11.046
- Zhao M, Liu F, Fu G, Zhang DZ, Zhang T, Zhou H. Improved mechanical properties and energy absorption of BCC lattice structures with triply periodic minimal surfaces fabricated by SLM. Materials. 2018;11(12):2411. doi: 10.3390/ma11122411
- Karageorgiou V, Kaplan D. Porosity of 3D biomaterial scaffolds and osteogenesis. Biomaterials. 2005;26(27): 5474–5491. doi: 10.1016/j.biomaterials.2005.02.002
- Han C, Li Y, Wang Q, et al. Continuous functionally graded porous titanium scaffolds manufactured by selective laser melting for bone implants. J Mech Behav Biomed Mater. 2018;80:119–127. doi: 10.1016/j.jmbbm.2018.01.013
- Wang S, Liu L, Li K, Zhu L, Chen J, Hao Y. Pore functionally graded Ti6Al4V scaffolds for bone tissue engineering application. Mater Des. 2019;168:107643. doi: 10.1016/j.matdes.2019.107643
- Lv Y, Guo J, Zhang Q, et al. Design of low elastic modulus and high strength TC4 porous scaffolds via new variable gradient strategies. Mater Lett. 2022;325:132616. doi: 10.1016/j.matlet.2022.132616
- Lv Y, Wang B, Liu G, et al. Metal material, properties and design methods of porous biomedical scaffolds for additive manufacturing: A review. Front Bioeng Biotechnol. 2021;9:641130. doi: 10.3389/fbioe.2021.641130
- Wang S, Shi Z, Liu L, Zhou X, Zhu L, Hao Yongqiang. The design of Ti6Al4V primitive surface structure with symmetrical gradient of pore size in biomimetic bone scaffold. Mater Des. 2020;193:108830. doi: 10.1016/j.matdes.2020.108830
- Ma S, Song K, Lan J, Ma L. Biological and mechanical property analysis for designed heterogeneous porous scaffolds based on the refined TPMS. J Mech Behav Biomed Mater. 2020;107:103727. doi: 10.1016/j.jmbbm.2020.103727
- Yánez A, Cuadrado A, Martel O, joão Afonso H, Monopoli D. Gyroid porous titanium structures: A versatile solution to be used as scaffolds in bone defect reconstruction. Mater Des. 2018;140:21–29. doi: 10.1016/j.matdes.2017.11.050
- Yoo D-J. Computer-aided porous scaffold design for tissue engineering using triply periodic minimal surfaces. Int J Precis Eng Manuf. 2011;12(1):61–71. doi: 10.1007/s12541-011-0008-9
- Fan Z, Fu Y, Gao R, Liu S. Investigation on heat transfer enhancement of phase change material for battery thermal energy storage system based on composite triply periodic minimal surface. J Energy Storage. 2023;57:106222. doi: 10.1016/j.est.2022.106222
- Yan C, Hao L, Hussein A, Young P. Ti–6Al–4V triply periodic minimal surface structures for bone implants fabricated via selective laser melting. J Mech Behav Biomed Mater. 2015;51:61–73. doi: 10.1016/j.jmbbm.2015.06.024
- Craeghs T, Clijsters S, Yasa E, Bechmann F, Berumen S, Kruth J-P. Determination of geometrical factors in layerwise laser melting using optical process monitoring. Opt Lasers Eng. 2011;49(12):1440–1446. doi: 10.1016/j.optlaseng.2011.06.016
- Li X, Xiong Y-Z, Zhang H, Gao R-N. Development of functionally graded porous titanium/silk fibroin composite scaffold for bone repair. Mater Lett. 2021;282:128670. doi: 10.1016/j.matlet.2020.128670
- Maconachie T, Leary M, Lozanovski B, et al. SLM lattice structures: Properties, performance, applications and challenges, Mater Des. 2019;183:108137. doi: 10.1016/j.matdes.2019.108137
- Tofail SAM, Koumoulos EP, Bandyopadhyay A, Bose S, O’Donoghue L, Charitidis C. Additive manufacturing: Scientific and technological challenges, market uptake and opportunities. Mater Today. 2018;21:22–37. doi: 10.1016/j.mattod.2017.07.001
- Lv Y, Wang B, Liu G, et al. Design of bone-like continuous gradient porous scaffold based on triply periodic minimal surfaces. J Mater Res Technol. 2022;21:3650–3665. doi: 10.1016/j.jmrt.2022.10.160
- Montazerian H, Davoodi E, Asadi-Eydivand M, Kadkhodapour J, Solati-Hashjin M. Porous scaffold internal architecture design based on minimal surfaces: A compromise between permeability and elastic properties. Mater Des. 2017;126:98–114. doi: 10.1016/j.matdes.2017.04.009
- Liu F, Mao Z, Zhang P, Zhang DZ, Jiang J, Ma Z. Functionally graded porous scaffolds in multiple patterns: New design method, physical and mechanical properties. Mater Des. 2018;160:849–860. doi: 10.1016/j.matdes.2018.09.053
- Yu G, Li Z, Li S, et al. The select of internal architecture for porous Ti alloy scaffold: A compromise between mechanical properties and permeability. Mater Des. 2020;192:108754. doi: 10.1016/j.matdes.2020.108754
- Cuadrado A, Yánez A, Martel O, Deviaene S, Monopoli D. Influence of load orientation and of types of loads on the mechanical properties of porous Ti6Al4V biomaterials. Mater Des. 2017;135:309–318. doi: 10.1016/j.matdes.2017.09.045
- Zhao S, Li SJ, Hou WT, Hao YL, Yang R, Misra RDK. The influence of cell morphology on the compressive fatigue behavior of Ti-6Al-4V meshes fabricated by electron beam melting. J Mech Behav Biomed Mater. 2016;59: 251–264. doi: 10.1016/j.jmbbm.2016.01.034
- Shi X, Yan C, Feng W, Zhang Y, Leng Z. Effect of high layer thickness on surface quality and defect behavior of Ti-6Al- 4V fabricated by selective laser melting. Opt Laser Technol. 2020;132:106471. doi: 10.1016/j.optlastec.2020.106471
- Ataee A, Li Y, Fraser D, Wen C, Song G. Anisotropic Ti- 6Al-4V gyroid scaffolds manufactured by electron beam melting (EBM) for bone implant applications. Mater Des. 2018;137:345–354. doi: 10.1016/j.matdes.2017.10.040