Challenges and advancements in high-throughput screening strategies for cancer therapeutics
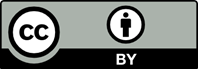
Drug discovery relies on high-throughput screening (HTS) methods incorporating both target- and cell-based assays. This comprehensive review delves into the challenges and benefits associated with these assays within the context of HTS. The strategies for developing screening assays, spanning both primary and secondary screens for target identification, are discussed. Furthermore, we review the methods of identifying the most efficacious drugs through these approaches for the treatment of cancer in detail. While various drugs have been identified for cancer treatment, there remains a pressing need for more relevant phenotypic assays. These assays aim to produce the desired disease phenotype, with a specific emphasis on highlighting targets rather than off-targets. The ultimate goal is to pave the way for innovative drug development strategies that can effectively treat cancer patients, thereby reducing the mortality rate.
- Macarron R, Banks MN, Bojanic D, et al. Impact of high-throughput screening in biomedical research. Nat Rev Drug Discov. 2011;10(3):188-195. doi: 10.1038/nrd3368
- Wigglesworth MJ, Murray DC, Blackett CJ, Kossenjans M, Nissink JW. Increasing the delivery of next generation therapeutics from high throughput screening libraries. Curr Opin Chem Biol. 2015;26:104-110. doi: 10.1016/j.cbpa.2015.04.006
- Pereira DA, Williams JA. Origin and evolution of high throughput screening. Br J Pharmacol. 2007;152(1):53-61. doi: 10.1038/sj.bjp.0707373
- Shun TY, Lazo JS, Sharlow ER, Johnston PA. Identifying actives from HTS data sets: Practical approaches for the selection of an appropriate HTS data-processing method and quality control review. J Biomol Screen. 2011;16(1):1-14. doi: 10.1177/1087057110389039
- Dreiman GHS, Bictash M, Fish PV, Griffin L, Svensson F. Changing the HTS paradigm: AI-driven iterative screening for hit finding. SLAS Discov. 2021;26(2):257-262. doi: 10.1177/2472555220949495
- Tsoli M, Wadham C, Pinese M, et al. Integration of genomics, high throughput drug screening, and personalized xenograft models as a novel precision medicine paradigm for high risk pediatric cancer. Cancer Biol Ther. 2018;19(12):1078-1087. doi: 10.1080/15384047.2018.1491498
- Mayr LM, Bojanic D. Novel trends in high-throughput screening. Curr Opin Pharmacol. 2009;9(5):580-588. doi: 10.1016/j.coph.2009.08.004
- Szymański P, Markowicz M, Mikiciuk-Olasik E. Adaptation of high-throughput screening in drug discovery-toxicological screening tests. Int J Mol Sci. 2012;13(1):427-452. doi: 10.3390/ijms13010427
- Milinkovic A, Martínez E. Nevirapine in the treatment of HIV. Expert Rev Anti Infect Ther. 2004;2(3):367-373. doi: 10.1586/14787210.2.3.367
- FDA. Approved Drugs Generated from High Throughput Screening Efforts I -20190719. Rome: FDA; 2019.
- Coussens NP, Braisted JC, Peryea T, Sittampalam GS, Simeonov A, Hall MD. Small-molecule screens: A gateway to cancer therapeutic agents with case studies of food and drug administration-approved drugs. Pharmacol Rev. 2017;69(4):479-496. doi: 10.1124/pr.117.013755
- Woodward AA, Urbanowicz RJ, Naj AC, Moore JH. Genetic heterogeneity: Challenges, impacts, and methods through an associative lens. Genet Epidemiol. 2022;46(8):555-571. doi: 10.1002/gepi.22497
- Lawson ARJ, Abascal F, Coorens THH, et al. Extensive heterogeneity in somatic mutation and selection in the human bladder. Science. 2020;370(6512):75-82. doi: 10.1126/science.aba8347
- Murphy N, Ward HA, Jenab M, et al. Heterogeneity of colorectal cancer risk factors by anatomical subsite in 10 European countries: A multinational cohort study. Clin Gastroenterol Hepatol. 2019;17(7):1323-1331.e6. doi: 10.1016/j.cgh.2018.07.030
- Haffner MC, Zwart W, Roudier MP, et al. Genomic and phenotypic heterogeneity in prostate cancer. Nat Rev Urol. 2021;18(2):79-92. doi: 10.1038/s41585-020-00400-w
- Mitelpunkt A, Galili T, Kozlovski T, et al. Novel Alzheimer’s disease subtypes identified using a data and knowledge driven strategy. Sci Rep. 2020;10(1):1327. doi: 10.1038/s41598-020-57785-2
- Margineanu DG. Systems biology, complexity, and the impact on antiepileptic drug discovery. Epilepsy Behav. 2014;38:131-142. doi: 10.1016/j.yebeh.2013.08.029
- Kell DB. Finding novel pharmaceuticals in the systems biology era using multiple effective drug targets, phenotypic screening and knowledge of transporters: where drug discovery went wrong and how to fix it. FEBS J. 2013;280(23):5957-5980. doi: 10.1111/febs.12268
- Ericson E, Gebbia M, Heisler LE, et al. Off-target effects of psychoactive drugs revealed by genome-wide assays in yeast. PLoS Genet. 2008;4(8):e1000151. doi: 10.1371/journal.pgen.1000151
- Lim H, Poleksic A, Yao Y, et al. Large-scale off-target identification using fast and accurate dual regularized one-class collaborative filtering and its application to drug repurposing. PLoS Comput Biol. 2016;12(10):e1005135. doi: 10.1371/journal.pcbi.1005135
- Lin A, Giuliano CJ, Palladino A, et al. Off-target toxicity is a common mechanism of action of cancer drugs undergoing clinical trials. Sci Transl Med. 2019;11(509):eaaw8412. doi: 10.1126/scitranslmed.aaw8412
- Koeberle A, Werz O. Multi-target approach for natural products in inflammation. Drug Discov Today. 2014;19(12):1871-1882. doi: 10.1016/j.drudis.2014.08.006
- Konecny GE, Pegram MD, Venkatesan N, et al. Activity of the dual kinase inhibitor lapatinib (GW572016) against HER-2-overexpressing and trastuzumab-treated breast cancer cells. Cancer Res. 2006;66(3):1630-1639. doi: 10.1158/0008-5472.Can-05-1182
- Flinn IW, O’Brien S, Kahl B, et al. Duvelisib, a novel oral dual inhibitor of PI3K-δ,γ, is clinically active in advanced hematologic malignancies. Blood. 2018;131(8):877-887. doi: 10.1182/blood-2017-05-786566
- Murai R, Yoshida Y, Muraguchi T, et al. A novel screen using the Reck tumor suppressor gene promoter detects both conventional and metastasis-suppressing anticancer drugs. Oncotarget. 2010;1(4):252-264.
- Herrmann IK, Wood MJA, Fuhrmann G. Extracellular vesicles as a next-generation drug delivery platform. Nat Nanotechnol. 2021;16(7):748-759. doi: 10.1038/s41565-021-00931-2
- Ohtaka H, Velázquez-Campoy A, Xie D, Freire E. Overcoming drug resistance in HIV-1 chemotherapy: The binding thermodynamics of Amprenavir and TMC-126 to wild-type and drug-resistant mutants of the HIV-1 protease. Protein Sci. 2002;11(8):1908-1916. doi: 10.1110/ps.0206402
- Wei F, Wang S, Gou X. A review for cell-based screening methods in drug discovery. Biophys Rep. 2021;7(6):504-516. doi: 10.52601/bpr.2021.210042
- Pathe-Neuschäfer-Rube A, Neuschäfer-Rube F, Püschel GP. Cell-based reporter release assay to determine the activity of calcium-dependent neurotoxins and neuroactive pharmaceuticals. Toxins (Basel). 2021;13(4):247. doi: 10.3390/toxins13040247
- Sanookpan K, Nonpanya N, Sritularak B, Chanvorachote P. Ovalitenone inhibits the migration of lung cancer cells via the suppression of AKT/mTOR and epithelial-to-mesenchymal transition. Molecules. 2021;26(3):638. doi: 10.3390/molecules26030638
- An WF, Tolliday N. Cell-based assays for high-throughput screening. Mol Biotechnol. 2010;45(2):180-186.
doi: 10.1007/s12033-010-9251-z
- Takenaka T. Classical vs reverse pharmacology in drug discovery. BJU Int. 2001;88 Suppl 2:7-10; discussion 49-50. doi: 10.1111/j.1464-410x.2001.00112.x
- Lage OM, Ramos MC, Calisto R, Almeida E, Vasconcelos V, Vicente F. Current screening methodologies in drug discovery for selected human diseases. Mar Drugs. 2018;16(8):279. doi: 10.3390/md16080279
- Sriram K, Insel PA. G Protein-coupled receptors as targets for approved drugs: How many targets and how many drugs? Mol Pharmacol. 2018;93(4):251-258. doi: 10.1124/mol.117.111062
- Kenny CH, Ding W, Kelleher K, et al. Development of a fluorescence polarization assay to screen for inhibitors of the FtsZ/ZipA interaction. Anal Biochem. 2003;323(2):224-233. doi: 10.1016/j.ab.2003.08.033
- Burns S, Travers J, Collins I, et al. Identification of small-molecule inhibitors of protein kinase B (PKB/AKT) in an AlphaScreen™ high-throughput screen. SLAS Discov. 2006;11(7):822-827. doi: 10.1177/1087057106290992
- Sudo K, Yamaji K, Kawamura K, et al. High-throughput screening of low molecular weight NS3-NS4A protease inhibitors using a fluorescence resonance energy transfer substrate. Antivir Chem Chemother. 2005;16(6):385-392. doi: 10.1177/095632020501600605
- Swaney S, McCroskey M, Shinabarger D, Wang Z, Turner BA, Parker CN. Characterization of a high-throughput screening assay for inhibitors of elongation factor P and ribosomal peptidyl transferase activity. J Biomol Screen. 2006;11(7):736-742. doi: 10.1177/1087057106291634
- Allen M, Reeves J, Mellor G. High throughput fluorescence polarization: A homogeneous alternative to radioligand binding for cell surface receptors. J Biomol Screen. 2000;5(2):63-69. doi: 10.1177/108705710000500202
- Xu J, Wang X, Ensign B, et al. Ion-channel assay technologies: Quo vadis? Drug Discov Today. 2001;6(24):1278-1287. doi: 10.1016/s1359-6446(01)02095-5
- Parker GJ, Law TL, Lenoch FJ, Bolger RE. Development of high throughput screening assays using fluorescence polarization: Nuclear receptor-ligand-binding and kinase/ phosphatase assays. J Biomol Screen. 2000;5(2):77-88. doi: 10.1177/108705710000500204
- Bagal SK, Brown AD, Cox PJ, et al. Ion channels as therapeutic targets: A drug discovery perspective. J Med Chem. 2013;56(3):593-624. doi: 10.1021/jm3011433
- Morachis JM, Huang R, Emerson BM. Identification of kinase inhibitors that target transcription initiation by RNA polymerase II. Oncotarget. 2011;2(1-2):18-28. doi: 10.18632/oncotarget.212
- Blay V, Tolani B, Ho SP, Arkin MR. High-throughput screening: Today’s biochemical and cell-based approaches. Drug Discov Today. 2020;25(10):1807-1821. doi: 10.1016/j.drudis.2020.07.024
- Glickman JF. Assay development for protein kinase enzymes. In: Markossian S, Grossman A, Brimacombe K, et al, editors. Assay Guidance Manual. United States: Eli Lilly & Company and the National Center for Advancing Translational Sciences; 2004.
- Harner MJ, Frank AO, Fesik SW. Fragment-based drug discovery using NMR spectroscopy. J Biomol NMR. 2013;56(2):65-75. doi: 10.1007/s10858-013-9740-z
- Bollag G, Hirth P, Tsai J, et al. Clinical efficacy of a RAF inhibitor needs broad target blockade in BRAF-mutant melanoma. Nature. 2010;467(7315):596-599. doi: 10.1038/nature09454
- Jadhav GP, Prathipati PK, Chauhan H. Surface plasmon resonance, Orbitrap mass spectrometry and Raman advancements: Exciting new techniques in drug discovery. Expert Opin Drug Discov. 2020;15(7):739-743. doi: 10.1080/17460441.2020.1745771
- Imaduwage KP, Lakbub J, Go EP, Desaire H. Rapid LC-MS based high-throughput screening method, affording no false positives or false negatives, identifies a new inhibitor for carbonic anhydrase. Sci Rep. 2017;7(1):10324. doi: 10.1038/s41598-017-08602-w
- McLaren DG, Shah V, Wisniewski T, et al. High-throughput mass spectrometry for hit identification: Current landscape and future perspectives. SLAS Discov. 2021;26(2):168-191. doi: 10.1177/2472555220980696
- Lu Y, Qin S, Zhang B, et al. Accelerating the throughput of affinity mass spectrometry-based ligand screening toward a G protein-coupled receptor. Anal Chem. 2019;91(13):8162-8169. doi: 10.1021/acs.analchem.9b00477
- Gilbert IH. Drug discovery for neglected diseases: Molecular target-based and phenotypic approaches. J Med Chem. 2013;56(20):7719-7726. doi: 10.1021/jm400362b
- Chen GQ, Xu Y, Shen SM, Zhang J. Phenotype and target-based chemical biology investigations in cancers. Natl Sci Rev. 2019;6(6):1111-1127. doi: 10.1093/nsr/nwy124
- Iqbal N, Iqbal N. Imatinib: A breakthrough of targeted therapy in cancer. Chemother Res Pract. 2014;2014:357027.
doi: 10.1155/2014/357027
- Rossari F, Minutolo F, Orciuolo E. Past, present, and future of Bcr-Abl inhibitors: From chemical development to clinical efficacy. J Hematol Oncol. 2018;11(1):84. doi: 10.1186/s13045-018-0624-2
- Dong B, Liang Z, Chen Z, et al. Cryptotanshinone suppresses key onco-proliferative and drug-resistant pathways of chronic myeloid leukemia by targeting STAT5 and STAT3 phosphorylation. Sci China Life Sci. 2018;61(9):999-1009. doi: 10.1007/s11427-018-9324-y
- Liu C, Nie D, Li J, et al. Antitumor effects of blocking protein neddylation in T315I-BCR-ABL leukemia cells and leukemia stem cells. Cancer Res. 2018;78(6):1522-1536. doi: 10.1158/0008-5472.Can-17-1733
- Misra S, Zhang X, Wani NA, Sizemore S, Ray A. Both BRCA1-wild type and -mutant triple-negative breast cancers show sensitivity to the NAE inhibitor MLN4924 which is enhanced upon MLN4924 and cisplatin combination treatment. Oncotarget. 2020;11(8):784-800. doi: 10.18632/oncotarget.27485
- Swinney DC, Lee JA. Recent advances in phenotypic drug discovery. F1000Res. 2020;9:F1000. doi: 10.12688/f1000research.25813.1
- McKinney JD, Richard A, Waller C, Newman MC, Gerberick F. The practice of structure activity relationships (SAR) in toxicology. Toxicol Sci. 2000;56(1):8-17. doi: 10.1093/toxsci/56.1.8
- Newman DJ, Cragg GM. Natural products as sources of new drugs over the nearly four decades from 01/1981 to 09/2019. J Nat Prod. 2020;83(3):770-803. doi: 10.1021/acs.jnatprod.9b01285
- Krejci P, Pejchalova K, Wilcox WR. Simple, mammalian cell-based assay for identification of inhibitors of the Erk MAP kinase pathway. Invest New Drugs. 2007;25(4):391-395. doi: 10.1007/s10637-007-9054-7
- Chambers C, Smith F, Williams C, et al. Measuring intracellular calcium fluxes in high throughput mode. Comb Chem High Throughput Screen. 2003;6(4):355-362. doi: 10.2174/138620703106298446
- Kariv I, Stevens ME, Behrens DL, Oldenburg KR. High throughput quantitation of cAMP production mediated by activation of seven transmembrane domain receptors. J Biomol Screen. 1999;4(1):27-32. doi: 10.1177/108705719900400105
- Eggert US, Kiger AA, Richter C, et al. Parallel chemical genetic and genome-wide RNAi screens identify cytokinesis inhibitors and targets. PLoS Biol. 2004;2(12):e379.
doi: 10.1371/journal.pbio.0020379
- Beck V, Pfitscher A, Jungbauer A. GFP-reporter for a high throughput assay to monitor estrogenic compounds. J Biochem Biophys Methods. 2005;64(1):19-37. doi: 10.1016/j.jbbm.2005.05.001
- Yarrow JC, Totsukawa G, Charras GT, Mitchison TJ. Screening for cell migration inhibitors via automated microscopy reveals a Rho-kinase inhibitor. Chem Biol. 2005;12(3):385-395. doi: 10.1016/j.chembiol.2005.01.015
- Vincent F, Loria P, Pregel M, et al. Developing predictive assays: The phenotypic screening “rule of 3”. Sci Transl Med. 2015;7(293):293ps15. doi: 10.1126/scitranslmed.aab1201
- Engle SJ, Vincent F. Small molecule screening in human induced pluripotent stem cell-derived terminal cell types. J Biol Chem. 2014;289(8):4562-4570. doi: 10.1074/jbc.R113.529156
- McNeish J, Roach M, Hambor J, et al. High-throughput screening in embryonic stem cell-derived neurons identifies potentiators of α-amino-3-hydroxyl-5-methyl-4- isoxazolepropionate-type glutamate receptors. J Biol Chem. 2010;285(22):17209-17217. doi: 10.1074/jbc.M109.098814
- Ashlock MA, Olson ER. Therapeutics development for cystic fibrosis: A successful model for a multisystem genetic disease. Annu Rev Med. 2011;62:107-125. doi: 10.1146/annurev-med-061509-131034
- Van Goor F, Hadida S, Grootenhuis PDJ, et al. Correction of the F508del-CFTR protein processing defect in vitro by the investigational drug VX-809. Proc Natl Acad Sci U S A. 2011;108(46):18843-18848. doi: 10.1073/pnas.1105787108
- Lee G, Ramirez CN, Kim H, et al. Large-scale screening using familial dysautonomia induced pluripotent stem cells identifies compounds that rescue IKBKAP expression. Nat Biotechnol. 2012;30(12):1244-1248. doi: 10.1038/nbt.2435
- Barberis A, Gunde T, Berset C, Audetat S, Lüthi U. Yeast as a screening tool. Drug Discov Today Technol. 2005;2(2):187-192. doi: 10.1016/j.ddtec.2005.05.022
- Balgi AD, Roberge M. Screening for chemical inhibitors of heterologous proteins expressed in yeast using a simple growth-restoration assay. Methods Mol Biol. 2009;486:125-137. doi: 10.1007/978-1-60327-545-3_9
- Puri AW, Bogyo M. Using small molecules to dissect mechanisms of microbial pathogenesis. ACS Chem Biol. 2009;4(8):603-616. doi: 10.1021/cb9001409
- Zlitni S, Blanchard JE, Brown ED. High-throughput screening of model bacteria. Methods Mol Biol. 2009;486:13-27.
- Hong CC. Large-scale small-molecule screen using zebrafish embryos. Methods Mol Biol. 2009;486:43-55. doi: 10.1007/978-1-60327-545-3_4
- Zon LI, Peterson RT. In vivo drug discovery in the zebrafish. Nat Rev Drug Discov. 2005;4(1):35-44. doi: 10.1038/nrd1606
- O’Rourke EJ, Conery AL, Moy TI. Whole-animal high-throughput screens: The C. elegans model. Methods Mol Biol. 2009;486:57-75. doi: 10.1007/978-1-60327-545-3_5
- Agee A, Carter D. Whole-organism screening: Plants. Methods Mol Biol. 2009;486:77-95. doi: 10.1007/978-1-60327-545-3_6
- Norambuena L, Raikhel NV, Hicks GR. Chemical genomics approaches in plant biology. Plant Syst Biol. 2009;553:345-354. doi: 10.1007/978-1-60327-563-7_18
- Stern HM, Murphey RD, Shepard JL, et al. Small molecules that delay S phase suppress a zebrafish bmyb mutant. Nat Chem Biol. 2005;1(7):366-370. doi: 10.1038/nchembio749
- Hong CC, Peterson QP, Hong JY, Peterson RT. Artery/vein specification is governed by opposing phosphatidylinositol-3 kinase and MAP kinase/ERK signaling. Curr Biol. 2006;16(13):1366-1372. doi: 10.1016/j.cub.2006.05.046
- Swinney DC. The value of translational biomarkers to phenotypic assays. Front Pharmacol. 2014;5:171. doi: 10.3389/fphar.2014.00171
- Ioannidis JP. Biomarker failures. Clin Chem. 2013;59(1):202-204. doi: 10.1373/clinchem.2012.185801
- Chau CH, Rixe O, McLeod H, Figg WD. Validation of analytic methods for biomarkers used in drug development. Clin Cancer Res. 2008;14(19):5967-5976. doi: 10.1158/1078-0432.Ccr-07-4535
- Spreafico R, Soriaga LB, Grosse J, Virgin HW, Telenti A. Advances in genomics for drug development. Genes (Basel). 2020;11(8):942. doi:10.3390/genes11080942
- Guo JB, Li XJ. Impacts of modern biology on drug discovery. Sheng Li Ke Xue Jin Zhan. 2007;38(1):25-31.
- Hong AL, Tseng YY, Cowley GS, et al. Integrated genetic and pharmacologic interrogation of rare cancers. Nat Commun. 2016;7:11987. doi: 10.1038/ncomms11987
- Lin X, Huang XP, Chen G, et al. Life beyond kinases: Structure-based discovery of sorafenib as nanomolar antagonist of 5-HT receptors. J Med Chem. 2012;55(12):5749-5759. doi: 10.1021/jm300338m
- Schneidewind T, Brause A, Pahl A, et al. Morphological profiling identifies a common mode of action for small molecules with different targets. Chembiochem. 2020;21(22):3197-3207. doi: 10.1002/cbic.202000381
- Han X, Zhou C, Jiang M, et al. Discovery of RG7834: The first-in-class selective and orally available small molecule hepatitis B virus expression inhibitor with novel mechanism of action. J Med Chem. 2018;61(23):10619-10634. doi: 10.1021/acs.jmedchem.8b01245
- Moffat JG, Vincent F, Lee JA, Eder J, Prunotto M. Opportunities and challenges in phenotypic drug discovery: An industry perspective. Nat Rev Drug Discov. 2017;16(8):531-543. doi: 10.1038/nrd.2017.111
- Vincent F, Loria PM, Weston AD, et al. Hit triage and validation in phenotypic screening: Considerations and strategies. Cell Chem Biol. 2020;27(11):1332-1346. doi: 10.1016/j.chembiol.2020.08.009
- Warchal SJ, Unciti-Broceta A, Carragher NO. Next-generation phenotypic screening. Future Med Chem. 2016;8(11):1331-1347. doi: 10.4155/fmc-2016-0025
- Comess KM, McLoughlin SM, Oyer JA, et al. Emerging approaches for the identification of protein targets of small molecules-a practitioners’ perspective. J Med Chem. 2018;61(19):8504-8535. doi: 10.1021/acs.jmedchem.7b01921
- Lee J, Bogyo M. Target deconvolution techniques in modern phenotypic profiling. Curr Opin Chem Biol. 2013;17(1):118-126. doi: 10.1016/j.cbpa.2012.12.022
- Lu JJ, Pan W, Hu YJ, Wang YT. Multi-target drugs: the trend of drug research and development. PLoS One. 2012;7(6):e40262. doi: 10.1371/journal.pone.0040262
- Rena G, Hardie DG, Pearson ER. The mechanisms of action of metformin. Diabetologia. 2017;60(9):1577-1585. doi: 10.1007/s00125-017-4342-z
- Keller MP, Attie AD. Physiological insights gained from gene expression analysis in obesity and diabetes. Annu Rev Nutr. 2010;30:341-364. doi: 10.1146/annurev.nutr.012809.104747
- Van’t Veer LJ, Bernards R. Enabling personalized cancer medicine through analysis of gene-expression patterns. Nature. 2008;452(7187):564-570. doi: 10.1038/nature06915
- Bertos NR, Park M. Breast cancer-one term, many entities? J Clin Invest. 2011;121(10):3789-3796.
doi: 10.1172/JCI57100
- Huang E, Ishida S, Pittman J, et al. Gene expression phenotypic models that predict the activity of oncogenic pathways. Nat Genet. 2003;34(2):226-230. doi: 10.1038/ng1167
- Loboda A, Nebozhyn M, Klinghoffer R, et al. A gene expression signature of RAS pathway dependence predicts response to PI3K and RAS pathway inhibitors and expands the population of RAS pathway activated tumors. BMC Med Genomics. 2010;3(1):26. doi: 10.1186/1755-8794-3-26
- Loboda A, Nebozhyn MV, Watters JW, et al. EMT is the dominant program in human colon cancer. BMC Med Genomics. 2011;4(1):9. doi: 10.1186/1755-8794-4-9
- Méndez-Lucio O, Naveja JJ, Vite-Caritino H, Prieto-Martínez FD, Medina-Franco JL. One drug for multiple targets: A computational perspective. J Mex Chem Soc. 2016;60(3):168-181. doi: 10.29356/jmcs.v60i3.100
- Hsieh JH, Smith-Roe SL, Huang R, et al. Identifying compounds with genotoxicity potential using Tox21 high-throughput screening assays. Chem Res Toxicol. 2019;32(7):1384-1401. doi: 10.1021/acs.chemrestox.9b00053
- Michod D, Widmann C. DNA-damage sensitizers: Potential new therapeutical tools to improve chemotherapy. Crit Rev Oncol Hematol. 2007;63(2):160-171. doi: 10.1016/j.critrevonc.2007.04.003
- Evans TJ, Yamamoto KN, Hirota K, Takeda S. Mutant cells defective in DNA repair pathways provide a sensitive high-throughput assay for genotoxicity. DNA Repair (Amst). 2010;9(12):1292-1298. doi: 10.1016/j.dnarep.2010.09.017
- Ji K, Kogame T, Choi K, et al. A novel approach using DNA-repair-deficient chicken DT40 cell lines for screening and characterizing the genotoxicity of environmental contaminants. Environ Health Perspect. 2009;117(11):1737-1744. doi: 10.1289/ehp.0900842
- Buerstedde JM, Takeda S. Increased ratio of targeted torandom integration after transfection of chicken B cell lines. Cell. 1991;67(1):179-188. doi: 10.1016/0092-8674(91)90581-i
- Yamazoe M, Sonoda E, Hochegger H, Takeda S. Reverse genetic studies of the DNA damage response in the chicken B lymphocyte line DT40. DNA Repair (Amst). 2004;3(8-9):1175-1185. doi: 10.1016/j.dnarep.2004.03.039
- Walmsley RM, Tate M. The GADD45a-GFP greenscreen HC assay. Methods Mol Biol. 2012;817:231-250. doi: 10.1007/978-1-61779-421-6_12
- Knight AW, Little S, Houck K, et al. Evaluation of high-throughput genotoxicity assays used in profiling the US EPA ToxCast™ chemicals. Regul Toxicol Pharmacol. 2009;55(2):188-199. doi: 10.1016/j.yrtph.2009.07.004
- Hastwell PW, Chai LL, Roberts KJ, et al. High-specificity and high-sensitivity genotoxicity assessment in a human cell line: Validation of the GreenScreen HC GADD45a-GFP genotoxicity assay. Mutat Res. 2006;607(2):160-175. doi: 10.1016/j.mrgentox.2006.04.011
- Giuliano KA, Gough AH, Taylor DL, Vernetti LA, Johnston PA. Early safety assessment using cellular systems biology yields insights into mechanisms of action. J Biomol Screen. 2010;15(7):783-797. doi: 10.1177/1087057110376413
- Brattain MG, Fine WD, Khaled FM, Thompson J, Brattain DE. Heterogeneity of malignant cells from a human colonic carcinoma. Cancer Res. 1981;41(5):1751-1756.
- Xing JZ, Zhu L, Gabos S, Xie L. Microelectronic cell sensor assay for detection of cytotoxicity and prediction of acute toxicity. Toxicol In Vitro. 2006;20(6):995-1004. doi: 10.1016/j.tiv.2005.12.008
- Fox JT, Sakamuru S, Huang R, et al. High-throughput genotoxicity assay identifies antioxidants as inducers of DNA damage response and cell death. Proc Natl Acad Sci. 2012;109(14):5423-5428. doi: 10.1073/pnas.1114278109
- Sikdar N, Banerjee S, Lee KY, et al. DNA damage responses by human ELG1 in S phase are important to maintain genomic integrity. Cell Cycle. 2009;8(19):3199-3207. doi: 10.4161/cc.8.19.9752
- Scholes DT, Banerjee M, Bowen B, Curcio MJ. Multiple regulators of Ty1 transposition in Saccharomyces cerevisiae have conserved roles in genome maintenance. Genetics. 2001;159(4):1449-1465. doi: 10.1093/genetics/159.4.1449
- Sykora P, Witt KL, Revanna P, et al. Next generation high throughput DNA damage detection platform for genotoxic compound screening. Sci Rep. 2018;8(1):2771. doi: 10.1038/s41598-018-20995-w
- Gatti L, Zunino F. Overview of tumor cell chemoresistance mechanisms. Methods Mol Med. 2005;111:127-148.
doi: 10.1385/1-59259-889-7:127
- Gottesman MM, Fojo T, Bates SE. Multidrug resistance in cancer: Role of ATP-dependent transporters. Nat Rev Cancer. 2002;2(1):48-58. doi: 10.1038/nrc706
- Gottesman MM, Ling V. The molecular basis of multidrug resistance in cancer: The early years of P-glycoprotein research. FEBS Lett. 2006;580(4):998-1009. doi: 10.1016/j.febslet.2005.12.060
- Sharom FJ. ABC multidrug transporters: Structure, function and role in chemoresistance. Pharmacogenomics. 2008;9(1):105-127. doi: 10.2217/14622416.9.1.105
- Sparreboom A, van Asperen J, Mayer U, et al. Limited oral bioavailability and active epithelial excretion of paclitaxel (Taxol) caused by P-glycoprotein in the intestine. Proc Natl Acad Sci U S A. 1997;94(5):2031-2035. doi: 10.1073/pnas.94.5.2031
- Kartner N, Riordan JR, Ling V. Cell surface P-glycoprotein associated with multidrug resistance in mammalian cell lines. Science. 1983;221(4617):1285-1288. doi: 10.1126/science.6137059
- Shen DW, Cardarelli C, Hwang J, et al. Multiple drug-resistant human KB carcinoma cells independently selected for high-level resistance to colchicine, adriamycin, or vinblastine show changes in expression of specific proteins. J Biol Chem. 1986;261(17):7762-7770.
- Roe M, Folkes A, Ashworth P, et al. Reversal of P-glycoprotein mediated multidrug resistance by novel anthranilamide derivatives. Bioorg Med Chem Lett. 1999;9(4):595-600. doi: 10.1016/s0960-894x(99)00030-x
- Dantzig AH, Shepard RL, Law KL, et al. Selectivity of the multidrug resistance modulator, LY335979, for P-glycoprotein and effect on cytochrome P-450 activities. J Pharmacol Exp Ther. 1999;290(2):854-862.
- Mistry P, Stewart AJ, Dangerfield W, et al. In vitro and in vivo reversal of P-glycoprotein-mediated multidrug resistance by a novel potent modulator, XR9576. Cancer Res. 2001;61(2):749-758.
- Bates SF, Chen C, Robey R, Kang M, Figg WD, Fojo T. Reversal of multidrug resistance: Lessons from clinical oncology. Novartis Found Symp. 2002;243:83-96; discussion 96-102, 180-185.
- Dalton W. Detection of multidrug resistance gene expression in multiple myeloma. Leukemia. 1997;11(7):1166-1169. doi: 10.1038/sj.leu.2400724
- Susa M, Choy E, Yang C, et al. Multidrug resistance reversal agent, NSC77037, identified with a cell-based screening assay. J Biomol Screen. 2010;15(3):287-296. doi: 10.1177/1087057109359422
- Winter SS, Lovato DM, Khawaja HM, et al. High-throughput screening for daunorubicin-mediated drug resistance identifies mometasone furoate as a novel ABCB1-reversal agent. J Biomol Screen. 2008;13(3):185-193. doi: 10.1177/1087057108314610
- Duan Z, Choy E, Hornicek FJ. NSC23925, identified in a high-throughput cell-based screen, reverses multidrug resistance. PLoS One. 2009;4(10):e7415. doi: 10.1371/journal.pone.0007415
- Ivnitski-Steele I, Larson RS, Lovato DM, et al. High-throughput flow cytometry to detect selective inhibitors of ABCB1, ABCC1, and ABCG2 transporters. Assay Drug Dev Technol. 2008;6(2):263-276. doi: 10.1089/adt.2007.107
- Gurdon JB, Elsdale TR, Fischberg M. Sexually mature individuals of Xenopus laevis from the transplantation of single somatic nuclei. Nature. 1958;182(4627):64-65. doi: 10.1038/182064a0
- Hass R, von der Ohe J, Ungefroren H. The intimate relationship among EMT, MET and TME: AT (ransdifferentiation) E (nhancing) M (ix) to be exploited for therapeutic purposes. Cancers. 2020;12(12):3674. doi: 10.3390/cancers12123674
- Saito S, Lin YC, Nakamura Y, et al. Potential application of cell reprogramming techniques for cancer research. Cell Mol Life Sci. 2019;76:45-65. doi: 10.1007/s00018-018-2924-7
- Welch DR. Tumor heterogeneity-a “contemporary concept” founded on historical insights and predictions. Cancer Res. 2016;76(1):4-6. doi: 10.1158/0008-5472.CAN-15-3024
- Denny SK, Yang D, Chuang CH, et al. Nfib promotes metastasis through a widespread increase in chromatin accessibility. Cell. 2016;166(2):328-342. doi: 10.1016/j.cell.2016.05.052
- Teng S, Li YE, Yang M, et al. Tissue-specific transcription reprogramming promotes liver metastasis of colorectal cancer. Cell Res. 2020;30(1):34-49. doi: 10.1038/s41422-019-0259-z
- Van Keymeulen A, Lee MY, Ousset M, et al. Reactivation of multipotency by oncogenic PIK3CA induces breast tumour heterogeneity. Nature. 2015;525(7567):119-123. doi: 10.1038/nature14665
- Katoh M. Canonical and non-canonical WNT signaling in cancer stem cells and their niches: Cellular heterogeneity, omics reprogramming, targeted therapy and tumor plasticity (Review). Int J Oncol. 2017;51(5):1357-1369. doi: 10.3892/ijo.2017.4129
- Zheng YW, Nie YZ, Taniguchi H. Cellular reprogramming and hepatocellular carcinoma development. World J Gastroenterol. 2013;19(47):8850-8860. doi: 10.3748/wjg.v19.i47.8850
- Jones PA, Laird PW. Cancer epigenetics comes of age. Nat Genet. 1999;21(2):163-167. doi: 10.1038/5947
- Wang D, Garcia-Bassets I, Benner C, et al. Reprogramming transcription by distinct classes of enhancers functionally defined by eRNA. Nature. 2011;474(7351):390-394. doi: 10.1038/nature10006
- Li HF, Keeton A, Vitolo M, et al. A high-throughput screen with isogenic PTEN+/+ and PTEN-/- cells identifies CID1340132 as a novel compound that induces apoptosis in PTEN and PIK3CA mutant human cancer cells. J Biomol Screen. 2011;16(4):383-393. doi: 10.1177/1087057110397357
- Qin L, Yin YT, Zheng FJ, et al. WNT5A promotes stemness characteristics in nasopharyngeal carcinoma cells leading to metastasis and tumorigenesis. Oncotarget. 2015;6(12):10239-10252. doi: 10.18632/oncotarget.3518
- Ewan K, Pajak B, Stubbs M, et al. A useful approach to identify novel small-molecule inhibitors of Wnt-dependent transcription. Cancer Res. 2010;70(14):5963-5973. doi: 10.1158/0008-5472.Can-10-1028
- Bialkowska AB, Yang VW. High-throughput screening strategies for targeted identification of therapeutic compounds in colorectal cancer. Future Oncol. 2012;8(3):259-272. doi: 10.2217/fon.12.19
- Lepourcelet M, Chen YNP, France DS, et al. Small-molecule antagonists of the oncogenic Tcf/β-catenin protein complex. Cancer Cell. 2004;5(1):91-102. doi: 10.1016/S1535-6108(03)00334-9
- Yu W, Ma Y, Shankar S, Srivastava RK. SATB2/β-catenin/ TCF-LEF pathway induces cellular transformation by generating cancer stem cells in colorectal cancer. Sci Rep. 2017;7(1):10939. doi: 10.1038/s41598-017-05458-y
- Torrance CJ, Agrawal V, Vogelstein B, Kinzler KW. Use of isogenic human cancer cells for high-throughput screening and drug discovery. Nat Biotechnol. 2001;19(10):940-945. doi: 10.1038/nbt1001-940
- Mallon R, Feldberg L, Kim S, et al. Identification of 4-anilino-3-quinolinecarbonitrile inhibitors of mitogen-activated protein/extracellular signal-regulated kinase 1 kinase. Mol Cancer Ther. 2004;3(6):755-762.
- Rissmann R, Hessel MH, Cohen AF. Vemurafenib/ dabrafenib and trametinib. Br J Clin Pharmacol. 2015;80(4):765-767. doi: 10.1111/bcp.12651
- Fu H, Gao H, Qi X, et al. Aldolase A promotes proliferation and G 1/S transition via the EGFR/MAPK pathway in non-small cell lung cancer. Cancer Commun (Lond). 2018;38:18. doi: 10.1186/s40880-018-0290-3
- Champer M, Miller D, Kuo DYS. Response to trametinib in recurrent low-grade serous ovarian cancer with NRAS mutation: A case report. Gynecol Oncol Rep. 2019;28:26-28. doi: 10.1016/j.gore.2019.01.007
- Liu S, Zou Q, Chen JP, et al. Targeting enhancer reprogramming to mitigate MEK inhibitor resistance in preclinical models of advanced ovarian cancer. J Clin Invest. 2021;131(20):e145035. doi: 10.1172/jci145035
- Yuliantie E, Dai X, Yang D, Crack PJ, Wang MW. High-throughput screening for small molecule inhibitors of the type-I interferon signaling pathway. Acta Pharm Sin B. 2018;8(6):889-899. doi: 10.1016/j.apsb.2018.07.005
- Dillon KJ, Smith GC, Martin NM. A FlashPlate assay for the identification of PARP-1 inhibitors. J Biomol Screen. 2003;8(3):347-352. doi: 10.1177/1087057103008003013
- Bapat A, Glass LS, Luo M, et al. Novel small-molecule inhibitor of apurinic/apyrimidinic endonuclease 1 blocks proliferation and reduces viability of glioblastoma cells. J Pharmacol Exp Ther. 2010;334(3):988-998. doi: 10.1124/jpet.110.169128
- Peterson EJ, Janzen WP, Kireev D, Singleton SF. High-throughput screening for RecA inhibitors using a transcreener adenosine 5’-O-diphosphate assay. Assay Drug Dev Technol. 2012;10(3):260-268. doi: 10.1089/adt.2011.0409
- Sexton JZ, Wigle TJ, He Q, et al. Novel inhibitors of E. coli RecA ATPase activity. Curr Chem Genomics. 2010;4:34-42. doi: 10.2174/1875397301004010034
- Huang F, Motlekar NA, Burgwin CM, Napper AD, Diamond SL, Mazin AV. Identification of specific inhibitors of human RAD51 recombinase using high-throughput screening. ACS Chem Biol. 2011;6(6):628-635. doi: 10.1021/cb100428c
- Huang L, Yi X, Yu X, et al. High-throughput strategies for the discovery of anticancer drugs by targeting transcriptional reprogramming. Front Oncol. 2021;11:762023. doi: 10.3389/fonc.2021.762023
- Shaw J, Dale I, Hemsley P, et al. Positioning high-throughput CETSA in early drug discovery through screening against B-Raf and PARP1. SLAS Discov. 2019;24(2):121-132. doi: 10.1177/2472555218813332
- Howard RT, Hemsley P, Petteruti P, et al. Structure-guided design and in-cell target profiling of a cell-active target engagement probe for PARP inhibitors. ACS Chem Biol. 2020;15(2):325-333. doi: 10.1021/acschembio.9b00963
- Cancer Genome Atlas Research Network. Comprehensive genomic characterization defines human glioblastoma genes and core pathways. Nature. 2008;455(7216):1061-1068. doi: 10.1038/nature07385
- Ding L, Getz G, Wheeler DA, et al. Somatic mutations affect key pathways in lung adenocarcinoma. Nature. 2008;455(7216):1069-1075. doi: 10.1038/nature07423
- Jones S, Zhang X, Parsons DW, et al. Core signaling pathways in human pancreatic cancers revealed by global genomic analyses. Science. 2008;321(5897):1801-1806. doi: 10.1126/science.1164368
- Steeg PS, Zhou Q. Cyclins and breast cancer. Breast Cancer Res Treat. 1998;52(1-3):17-28. doi: 10.1023/a:1006102916060
- Miyoshi Y, Iwao K, Egawa C, Noguchi S. Association of centrosomal kinase STK15/BTAK mRNA expression with chromosomal instability in human breast cancers. Int J Cancer. 2001;92(3):370-373. doi: 10.1002/ijc.1200
- Dhillon NK, Mudryj M. Cyclin E overexpression enhances cytokine-mediated apoptosis in MCF7 breast cancer cells. Genes Immun. 2003;4(5):336-342. doi: 10.1038/sj.gene.6363973
- Niu D, Wang G, Wang X. Up-regulation of cyclin E in breast cancer via estrogen receptor pathway. Int J Clin Exp Med. 2015;8(1):910-915.
- Warner SL, Munoz RM, Stafford P, et al. Comparing Aurora A and Aurora B as molecular targets for growth inhibition of pancreatic cancer cells. Mol Cancer Ther. 2006;5(10):2450-2458. doi: 10.1158/1535-7163.Mct-06-0202
- Wang W, Kim SH, El-Deiry WS. Small-molecule modulators of p53 family signaling and antitumor effects in p53-deficient human colon tumor xenografts. Proc Natl Acad Sci U S A. 2006;103(29):11003-11008. doi: 10.1073/pnas.0604507103
- Maloney A, Workman P. HSP90 as a new therapeutic target for cancer therapy: The story unfolds. Expert Opin Biol Ther. 2002;2(1):3-24. doi: 10.1517/14712598.2.1.3
- Magwenyane AM, Ugbaja SC, Amoako DG, Somboro AM, Khan RB, Kumalo HM. Heat shock protein 90 (HSP90) inhibitors as anticancer medicines: A review on the computer-aided drug discovery approaches over the past five years. Comput Math Methods Med. 2022;2022:2147763. doi: 10.1155/2022/2147763
- Bos R, Zhong H, Hanrahan CF, et al. Levels of hypoxia-inducible factor-1 alpha during breast carcinogenesis. J Natl Cancer Inst. 2001;93(4):309-314. doi: 10.1093/jnci/93.4.309
- Semenza GL. HIF-1 and tumor progression: Pathophysiology and therapeutics. Trends Mol Med. 2002;8(4 Suppl):S62-S67. doi: 10.1016/s1471-4914(02)02317-1
- Masoud GN, Li W. HIF-1α pathway: Role, regulation and intervention for cancer therapy. Acta Pharm Sin B. 2015;5(5):378-389. doi: 10.1016/j.apsb.2015.05.007
- Workman P. Scoring a bull’s-eye against cancer genome targets. Curr Opin Pharmacol. 2001;1(4):342-352. doi: 10.1016/s1471-4892(01)00060-1
- Mahlknecht U, Hoelzer D. Histone acetylation modifiers in the pathogenesis of malignant disease. Mol Med. 2000;6(8):623-644.
- Turlais F, Hardcastle A, Rowlands M, et al. High-throughput screening for identification of small molecule inhibitors of histone acetyltransferases using scintillating microplates (FlashPlate). Anal Biochem. 2001;298(1):62-68. doi: 10.1006/abio.2001.5340
- Dekker FJ, Haisma HJ. Histone acetyl transferases as emerging drug targets. Drug Discov Today. 2009;14(19-20):942-948. doi: 10.1016/j.drudis.2009.06.008
- Miles DW. Update on HER-2 as a target for cancer therapy: Herceptin in the clinical setting. Breast Cancer Res. 2001;3(6):380-384. doi: 10.1186/bcr326
- Kelland LR, Smith V, Valenti M, et al. Preclinical antitumor activity and pharmacodynamic studies with the farnesyl protein transferase inhibitor R115777 in human breast cancer. Clin Cancer Res. 2001;7(11):3544-3550.
- Johnston SR. Farnesyl transferase inhibitors: A novel targeted tnerapy for cancer. Lancet Oncol. 2001;2(1):18-26. doi: 10.1016/s1470-2045(00)00191-1
- Nicholls H. Aromatase inhibitors continue their ATAC on tamoxifen. Trends Mol Med. 2002;8(4 Suppl):S12-S13. doi: 10.1016/s1471-4914(02)02304-3
- Buzdar AU. Anastrozole (Arimidex)--an aromatase inhibitor for the adjuvant setting? Br J Cancer. 2001;85 Suppl 2(Suppl 2):6-10. doi: 10.1054/bjoc.2001.1983
- Chen B, Dodge ME, Tang W, et al. Small molecule-mediated disruption of Wnt-dependent signaling in tissue regeneration and cancer. Nat Chem Biol. 2009;5(2):100-107. doi: 10.1038/nchembio.137
- Zhang N, Wu B, Powell D, et al. Synthesis and structure-activity relationships of 3-cyano-4-(phenoxyanilino) quinolines as MEK (MAPKK) inhibitors. Bioorg Med Chem Lett. 2000;10(24):2825-2828. doi: 10.1016/S0960-894X(00)00580-1
- Berger D, Dutia M, Powell D, et al. Synthesis and evaluation of 4-anilino-6,7-dialkoxy-3-quinolinecarbonitriles as inhibitors of kinases of the Ras-MAPK signaling cascade. Bioorg Med Chem Lett. 2003;13(18):3031-3034. doi: 10.1016/s0960-894x(03)00640-1
- Fry DW, Kraker AJ, McMichael A, et al. A specific inhibitor of the epidermal growth factor receptor tyrosine kinase. Science. 1994;265(5175):1093-1095. doi: 10.1126/science.8066447