Relationship Between Winds with Surface Roughness and Carbon Dioxide Concentrations Over Iraq
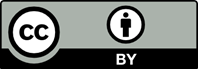
The methodology used in the study is based on hourly and monthly rates (wind speed and direction, CO2, and surface roughness) obtained from the European Center for Numerical Weather Prediction at 30 sites in Iraq in 2020. The results showed that the maximum wind speed was 4 m/s at 12:00 noon, while the prevailing wind direction for all sites in Iraq was towards the northwest (NW) and the minimum wind speed was 2 m/s at 00:00 AM. By analysing the monthly wind speed and direction for some selected stations, it was found that the highest value of (SW), i.e., 64% was recorded at Rutba station, and the lowest value of (SW) at Basra station was 45%, where the prevailing direction was found to be towards the north-northwest (NNW). The spatial analysis concluded that the wind movement is directed from the north and northwest, explaining that the wind reverses its direction from the mountainous heights to flat lands due to the roughness of the surface in the northern regions above the stations of Iraq. Spearman’s test was carried out between wind speed and surface roughness, and between carbon dioxide and surface roughness. It was found that the correlation strength is weak, and the relationship is inverse between surface roughness and wind speed. This analysis is considered the best way to choose the best wind power plants.
Abbassi, Y., Ahmadikia, H. and E. Baniasadi (2022). Impact of wind speed on urban heat and pollution islands. Urban Climate, 44: 101200.
Abbood, Z.M., Al-Taai, O.T. and W.G. Nassif (2021). Impact of wind speed and direction on low cloud cover over Baghdad city. Current Applied Science and Technology, 21(3): 590-600.
Al-Taai, O.T., Abbood, Z.M. and J.H. Kadhum (2021). Determination stability potential energy of thunderstorms for some severe weather forecasting cases in Baghdad city. Journal of Green Engineering,11(1): 779-794.
Alzubaidi, M., Hasan, K.N. and M. Lasantha (2022). Impact of probabilistic modeling of wind speed on power system voltage profile and voltage stability analysis. Electric Power Systems Research, 206: 107807.
Csavina, Janae, Jason Field, Omar Félix, Corral-Avitia, A.Y., Sáez, A.E. and E.A. Betterton (2014). Effect of wind speed and relative humidity on atmospheric dust concentrations in semi-arid climates. Science of the Total Environment, 487: 82-90.
Dadaser‐Celik, F. and E. Cengiz (2014). Wind speed trends over Turkey from 1975 to 2006. International Journal of Climatology, 34(6): 1913-1927.
Ehrmann, R.S., Wilcox, B., White, E.B. and D.C. Maniaci (2017). Effect of surface roughness on wind turbine performance.No. SAND-2017-10669. Sandia National Lab. (SNL-NM), Albuquerque, NM (United States).
Laban, O.N., Maghanga, C.M. and K. Joash (2019). Determination of the surface roughness parameter and wind shear exponent of Kisii region from the on-site measurement of wind profiles. Journal of Energy, 2019: 8264061.
Lee, D., Jeong, S.Y. and T.H.K. Kang (2022). Consideration of terrain features from satellite imagery in machine learning of basic wind speed. Building and Environment, 213: 108866.
Liu, X., Kang, Y., Chen, H. and H. Lu (2022). Comparison of surface wind speed and wind speed profiles in the Taklimakan Desert. Peer J., 10: e13001.
López, G. and P. Arboleya (2022). Short-term wind speed forecasting over complex terrain using linear regression models and multivariable LSTM and NARX networks in the Andes Mountains, Ecuador. Renewable Energy, 183: 351-368.
Özkan, M. and O. Erkan (2022). Control of a boundary layer over a wind turbine blade using distributed passive roughness. Renewable Energy, 184: 421-429.
Ro, K.S. and P.G. Hunt (2007). Characteristic wind speed distributions and reliability of the logarithmic wind profile. Journal of environmental engineering, 133(3): 313-318.
Souza, N.B.P., Nascimento, E.G.S. and D.M. Moreira (2023). Performance evaluation of the WRF model in a tropical region: Wind speed analysis at different sites. Atmósfera, 36(2): 253-277.
Sun, F. and J. Tongdan (2022). A hybrid approach to multistep, short-term wind speed forecasting using correlated features. Renewable Energy, 186: 742-754.
Tamalouzt, S., Belkhier, Y.,Sahri, Y., Ullah, N., Shaw, R.N. and M. Bajaj (2022). New direct reactive power control based fuzzy and modulated hysteresis method for micro-grid applications under real wind speed. Energy Sources, Part A: Recovery, Utilization, and Environmental Effects, 44(2): 4862-4887.
The European Centre for Medium-Range Weather Forecasts (2020). ECMWF Overview. https://www.ecmwf.int/en/forecasts/datasets.
Wever, N. (2012). Quantifying trends in surface roughness and the effect on surface wind speed observations. Journal of Geophysical Research: Atmospheres, 117: 11.
Wilson, T.C., Brenner, J., Morrison, Z., Jacob, J.D. and B.R. Elbing (2022). Wind speed statistics from a small UAS and its sensitivity to the sensor location. Atmosphere, 13(3): 443.
Yang, D., Wang, W. and T. Hong (2022). A historical weather forecast dataset from the European Centre for Medium-Range Weather Forecasts (ECMWF) for energy forecasting. Solar Energy, 232: 263-274.
Yehia, M.A., Al-Taai, O.T. and M.K. Ibrahim (2023). Spatiotemporal Distribution of Mean Temperature and Total Precipitation over Iraq for a Period (1980-2017). IOP Conference Series: Earth and Environmental Science, 1158(3): 032004.
Zhang, H., Mingwei, Ge., Liu, Y. and X.I.A. Yang (2021). A new coupled model for the equivalent roughness heights of wind farms. Renewable Energy, 171: 34-46.