Generation of vascularized brain organoids: Technology, applications, and prospects
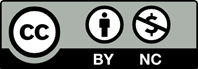
Brain organoids have become important tools for studying neural development and disease modeling by closely mimicking in vivo brain architecture and function. However, despite their potential, conventional brain organoids lack a vascular system, limiting their physiological relevance and growth due to restricted oxygen and nutrient supply. Recent advances in generating vascularized brain organoids (V-Organoids) have sought to overcome such limitations, allowing for more accurate modeling of human brain development, neurovascular interactions, and blood– brain barrier (BBB) function. This article provides a comprehensive review of the methodologies employed to enhance the vascularization of brain organoids, including induction techniques, biomaterials, advanced bioengineering approaches, and in vivo implantation. The introduction of functional vasculature into brain organoids has not only enhanced their survival and maturation but also expanded their utility in disease modeling, drug screening, and regenerative medicine. We also discuss the applications of V-Organoids in the study of neurodevelopmental processes, BBB permeability in neurological disorders, brain cancer, and regeneration applications. Despite significant progress made in the development of V-Organoids, challenges such as vascular maturity, immune integration, longevity, and ethical considerations must be addressed to enable more accurate brain models, which could enhance our understanding of neurodevelopment and potential treatments.
- Clarke JM. Neuroanatomy: Brain structure and function. Neuropsychology. Amsterdam, Netherlands: Elsevier; 1994. p. 29-52.
- Sousa AMM, Meyer KA, Santpere G, Gulden FO, Sestan N. Evolution of the human nervous system function, structure, and development. Cell. 2017;170(2):226-247. doi: 10.1016/j.cell.2017.06.036
- Farah MJ. Neuroethics: The ethical, legal, and societal impact of neuroscience. Annu Rev Psychol. 2012;63(1):571-591. doi: 10.1146/annurev.psych.093008.100438
- Lancaster MA, Renner M, Martin CA, et al. Cerebral organoids model human brain development and microcephaly. Nature. 2013;501(7467):373-379. doi: 10.1038/nature12517
- Qian X, Nguyen HN, Song MM, et al. Brain-region-specific organoids using mini-bioreactors for modeling ZIKV exposure. Cell. 2016;165(5):1238-1254. doi: 10.1016/j.cell.2016.04.032
- Pasca AM, Sloan SA, Clarke LE, et al. Functional cortical neurons and astrocytes from human pluripotent stem cells in 3D culture. Nat Methods. 2015;12(7):671-678. doi: 10.1038/nmeth.3415
- Molnár Z, Clowry GJ, Šestan N, et al. New insights into the development of the human cerebral cortex. J Anat. 2019;235(3):432-451. doi: 10.1111/joa.13055
- Camp JG, Badsha F, Florio M, et al. Human cerebral organoids recapitulate gene expression programs of fetal neocortex development. Proc Natl Acad Sci U S A. 2015;112(51):15672-15677. doi: 10.1073/pnas.1520760112
- Di Lullo E, Kriegstein AR. The use of brain organoids to investigate neural development and disease. Nat Rev Neurosci. 2017;18(10):573-584. doi: 10.1038/nrn.2017.107
- Bose R, Banerjee S, Dunbar GL. Modeling neurological disorders in 3D organoids using human-derived pluripotent stem cells. Front Cell Dev Biol. 2021;9:640212. doi: 10.3389/fcell.2021.640212
- Cao Z, Kong F, Ding J, Chen C, He F, Deng W. Promoting Alzheimer’s disease research and therapy with stem cell technology. Stem Cell Res Ther. 2024;15(1):136. doi: 10.1186/s13287-024-03737-w
- Trujillo CA, Muotri AR. Brain organoids and the study of neurodevelopment. Trends Mol Med. 2018;24(12):982-990. doi: 10.1016/j.molmed.2018.09.005
- Cao SY, Yang D, Huang ZQ, et al. Cerebral organoids transplantation repairs infarcted cortex and restores impaired function after stroke. NPJ Regen Med. 2023;8(1):27. doi: 10.1038/s41536-023-00301-7
- Dong X, Xu SB, Chen X, et al. Human cerebral organoids establish subcortical projections in the mouse brain after transplantation. Mol Psychiatry. 2021;26(7):2964-2976. doi: 10.1038/s41380-020-00910-4
- Kelava I, Lancaster MA. Dishing out mini-brains: Current progress and future prospects in brain organoid research. Dev Biol. 2016;420(2):199-209. doi: 10.1016/j.ydbio.2016.06.037
- Matsui TK, Tsuru Y, Hasegawa K, Kuwako KI. Vascularization of human brain organoids. Stem Cells. 2021;39(8):1017-1024. doi: 10.1002/stem.3368
- Cakir B, Xiang Y, Tanaka Y, et al. Engineering of human brain organoids with a functional vascular-like system. Nat Methods. 2019;16(11):1169-1175. doi: 10.1038/s41592-019-0586-5
- Pham MT, Pollock KM, Rose MD, et al. Generation of human vascularized brain organoids. Neuroreport. 2018;29(7):588-593. doi: 10.1097/WNR.0000000000001014
- Giandomenico SL, Mierau SB, Gibbons GM, et al. Cerebral organoids at the air-liquid interface generate diverse nerve tracts with functional output. Nat Neurosci. 2019;22(4):669-679. doi: 10.1038/s41593-019-0350-2
- Wang H. Modeling neurological diseases with human brain organoids. Front Synaptic Neurosci. 2018;10:15. doi: 10.3389/fnsyn.2018.00015
- Sweeney MD, Zhao Z, Montagne A, Nelson AR, Zlokovic BV. Blood-brain barrier: From physiology to disease and back. Physiol Rev. 2019;99:21-78. doi: 10.1152/physrev.00050.2017
- Sun XY, Ju XC, Li Y, et al. Generation of vascularized brain organoids to study neurovascular interactions. Elife. 2022;11:e76707. doi: 10.7554/eLife.76707
- Lancaster MA. Brain organoids get vascularized. Nat Biotechnol. 2018;36(5):407-408. doi: 10.1038/nbt.4133
- Sun XY, Ju XC, Zhao HF, You ZW, Han RR, Luo ZG. Generation of human blood vessel and vascularized cerebral organoids. Bio Protoc. 2023;13(21):e4870. doi: 10.21769/BioProtoc.4870
- Zhu Y, Sun L, Fu X, et al. Engineering microcapsules to construct vascularized human brain organoids. Chem Eng J. 2021;424:130427. doi: 10.1016/j.cej.2021.130427
- Ye B. Approaches to vascularizing human brain organoids. PLoS Biol. 2023;21(5):e3002141. doi: 10.1371/journal.pbio.3002141
- Sun X, Kofman S, Ogbolu VC, Karch CM, Ibric L, Qiang L. Vascularized brain assembloids with enhanced cellular complexity provide insights into the cellular deficits of tauopathy. Stem Cells. 2024;42(2):107-115. doi: 10.1093/stmcls/sxad086
- Kolesky DB, Truby RL, Gladman AS, Busbee TA, Homan KA, Lewis JA. 3D bioprinting of vascularized, heterogeneous cell-laden tissue constructs. Adv Mater. 2014;26(19):3124-3130. doi: 10.1002/adma.201305506
- Nieuwenhuys R, Voogd J, Van Huijzen C. The Human Central Nervous System: A Synopsis and Atlas. Berlin, Germany: Springer Science & Business Media; 2007.
- Kandel ER, Schwartz JH, Jessell TM, Siegelbaum S, Hudspeth AJ, Mack S. Principles of Neural Science. Vol. 4. New York: McGraw-Hill; 2000.
- Mountcastle VB. The columnar organization of the neocortex. Brain. 1997;120(Pt 4):701-722. doi: 10.1093/brain/120.4.701
- Gazzaniga M, Ivry R, Mangun G. Cognitive Neuroscience: The Biology of the Mind. 5th ed. New York: WW Norton & Company; 2018.
- Clark DL, Boutros NN, Mendez MF. The Brain and Behavior: An Introduction to Behavioral Neuroanatomy. United Kingdom: Cambridge University Press; 2010.
- Von Economo C. Cellular Structure of the Human Cerebral Cortex. Basel, Switzerland: Karger Medical and Scientific Publishers; 2009.
- Tambalo M, Lodato S. Brain organoids: Human 3D models to investigate neuronal circuits assembly, function and dysfunction. Brain Res. 2020;1746:147028. doi: 10.1016/j.brainres.2020.147028
- Rakic P. Evolution of the neocortex: A perspective from developmental biology. Nat Rev Neurosci. 2009;10(10):724-735. doi: 10.1038/nrn2719
- Miller EK, Cohen JD. An integrative theory of prefrontal cortex function. Annu Rev Neurosci. 2001;24(1):167-202. doi: 10.1146/annurev.neuro.24.1.167
- Carmeliet P, Tessier-Lavigne M. Common mechanisms of nerve and blood vessel wiring. Nature. 2005;436(7048):193-200. doi: 10.1038/nature03875
- Hanahan D, Folkman J. Patterns and emerging mechanisms of the angiogenic switch during tumorigenesis. Cell. 1996;86(3):353-364. doi: 10.1016/s0092-8674(00)80108-7
- Ruhrberg C, Bautch VL. Neurovascular development and links to disease. Cell Mol Life Sci. 2013;70(10):1675-1684. doi: 10.1007/s00018-013-1277-5
- Vasudevan A, Bhide PG. Angiogenesis in the embryonic CNS: A new twist on an old tale. Cell Adh Migr. 2008;2(3):167-169. doi: 10.4161/cam.2.3.6485
- Walchli T, Bisschop J, Carmeliet P, et al. Shaping the brain vasculature in development and disease in the single-cell era. Nat Rev Neurosci. 2023;24(5):271-298. doi: 10.1038/s41583-023-00684-y
- Augustin HG, Young Koh G, Thurston G, Alitalo K. Control of vascular morphogenesis and homeostasis through the angiopoietin-Tie system. Nat Rev Mol Cell Biol. 2009;10(3):165-177. doi: 10.1038/nrm2639
- Ransohoff RM. How neuroinflammation contributes to neurodegeneration. Science. 2016;353(6301):777-783. doi: 10.1126/science.aag2590
- Blanco R, Gerhardt H. VEGF and Notch in tip and stalk cell selection. Cold Spring Harb Perspect Med. 2013;3(1):a006569. doi: 10.1101/cshperspect.a006569
- Yang Y, Torbey MT. Angiogenesis and blood-brain barrier permeability in vascular remodeling after stroke. Curr Neuropharmacol. 2020;18(12):1250-1265. doi: 10.2174/1570159X18666200720173316
- Patan S. Vasculogenesis and angiogenesis. Cancer Treat Res. 2004;117:3-32. doi: 10.1007/978-1-4419-8871-3_1
- Zeng A, Wang SR, He YX, Yan Y, Zhang Y. Progress in understanding of the stalk and tip cells formation involvement in angiogenesis mechanisms. Tissue Cell. 2021;73:101626. doi: 10.1016/j.tice.2021.101626
- Omorphos NP, Gao C, Tan SS, Sangha MS. Understanding angiogenesis and the role of angiogenic growth factors in the vascularisation of engineered tissues. Mol Biol Rep. 2021;48(1):941-950. doi: 10.1007/s11033-020-06108-9
- Herbert SP, Stainier DY. Molecular control of endothelial cell behaviour during blood vessel morphogenesis. Nat Rev Mol Cell Biol. 2011;12(9):551-564. doi: 10.1038/nrm3176
- Potente M, Carmeliet P. The link between angiogenesis and endothelial metabolism. Annu Rev Physiol. 2017;79(1):43-66. doi: 10.1146/annurev-physiol-021115-105134
- Liu S, Agalliu D, Yu C, Fisher M. The role of pericytes in blood-brain barrier function and stroke. Curr Pharm Des. 2012;18(25):3653-3662. doi: 10.2174/138161212802002706
- Lemaitre V, D’Armiento J. Matrix metalloproteinases in development and disease. Birth Defects Res C Embryo Today. 2006;78(1):1-10. doi: 10.1002/bdrc.20065
- Jain RK. Normalization of tumor vasculature: An emerging concept in antiangiogenic therapy. Science. 2005;307(5706):58-62. doi: 10.1126/science.1104819
- Armulik A, Genove G, Betsholtz C. Pericytes: Developmental, physiological, and pathological perspectives, problems, and promises. Dev Cell. 2011;21(2):193-215. doi: 10.1016/j.devcel.2011.07.001
- Rattner A, Wang Y, Nathans J. Signaling pathways in neurovascular development. Annu Rev Neurosci. 2022;45(1):87-108. doi: 10.1146/annurev-neuro-111020-102127
- Gupta A, Rarick KR, Ramchandran R. Established, new and emerging concepts in brain vascular development. Front Physiol. 2021;12:636736. doi: 10.3389/fphys.2021.636736
- Wang Q, Huang X, Su Y, et al. Activation of Wnt/β-catenin pathway mitigates blood-brain barrier dysfunction in Alzheimer’s disease. Brain. 2022;145(12):4474-4488. doi: 10.1093/brain/awac236
- Ma J, Sanchez-Duffhues G, Goumans MJ, Ten Dijke P. TGF- β-induced endothelial to mesenchymal transition in disease and tissue engineering. Front Cell Dev Biol. 2020;8:260. doi: 10.3389/fcell.2020.00260
- Cash A, Theus MH. Mechanisms of blood-brain barrier dysfunction in traumatic brain injury. Int J Mol Sci. 2020;21(9):3344. doi: 10.3390/ijms21093344
- Aldape K, Brindle KM, Chesler L, et al. Challenges to curing primary brain tumours. Nat Rev Clin Oncol. 2019;16(8):509-520. doi: 10.1038/s41571-019-0177-5
- Carmeliet P, Jain RK. Principles and mechanisms of vessel normalization for cancer and other angiogenic diseases. Nat Rev Drug Discov. 2011;10(6):417-427. doi: 10.1038/nrd3455
- Abbott NJ, Friedman A. Overview and introduction: The blood-brain barrier in health and disease. Epilepsia. 2012;53(Suppl 6):1-6. doi: 10.1111/j.1528-1167.2012.03696.x
- Sadanandan J, Thomas S, Mathew IE, et al. Establishing and maintaining the blood-brain barrier: Epigenetic and signaling determinants. bioRxiv. 2022:516809. doi: 10.1101/2022.11.29.516809
- Obermeier B, Daneman R, Ransohoff RM. Development, maintenance and disruption of the blood-brain barrier. Nat Med. 2013;19(12):1584-1596. doi: 10.1038/nm.3407
- Daneman R, Prat A. The blood-brain barrier. Cold Spring Harb Perspect Biol. 2015;7(1):a020412. doi: 10.1101/cshperspect.a020412
- Hladky SB, Barrand MA. Mechanisms of fluid movement into, through and out of the brain: evaluation of the evidence. Fluids Barriers CNS. 2014;11:26. doi: 10.1186/2045-8118-11-26
- Iadecola C. The neurovascular unit coming of age: A journey through neurovascular coupling in health and disease. Neuron. 2017;96(1):17-42. doi: 10.1016/j.neuron.2017.07.030
- Varatharaj A, Galea I. The blood-brain barrier in systemic inflammation. Brain Behav Immun. 2017;60:1-12. doi: 10.1016/j.bbi.2016.03.010
- Zlokovic BV. Neurovascular pathways to neurodegeneration in Alzheimer’s disease and other disorders. Nat Rev Neurosci. 2011;12(12):723-738. doi: 10.1038/nrn3114
- Engelhardt B, Ransohoff RM. The ins and outs of T-lymphocyte trafficking to the CNS: Anatomical sites and molecular mechanisms. Trends Immunol. 2005;26(9):485-495. doi: 10.1016/j.it.2005.07.004
- Sweeney MD, Sagare AP, Zlokovic BV. Blood-brain barrier breakdown in Alzheimer disease and other neurodegenerative disorders. Nat Rev Neurol. 2018;14(3):133-150. doi: 10.1038/nrneurol.2017.188
- Yang Y, Rosenberg GA. Blood-brain barrier breakdown in acute and chronic cerebrovascular disease. Stroke. 2011;42(11):3323-3328. doi: 10.1161/STROKEAHA.110.608257
- Daneman R. The blood-brain barrier in health and disease. Ann Neurol. 2012;72(5):648-672. doi: 10.1002/ana.23648
- Keaney J, Campbell M. The dynamic blood-brain barrier. FEBS J. 2015;282(21):4067-4079. doi: 10.1111/febs.13412
- Abbott NJ, Pizzo ME, Preston JE, Janigro D, Thorne RG. The role of brain barriers in fluid movement in the CNS: Is there a ‘glymphatic’system? Acta Neuropathol. 2018;135:387-407. doi: 10.1007/s00401-018-1812-4
- Muoio V, Persson PB, Sendeski MM. The neurovascular unit - concept review. Acta Physiol (Oxf). 2014;210(4):790-798. doi: 10.1111/apha.12250
- Mansour AA, Schafer ST, Gage FH. Cellular complexity in brain organoids: Current progress and unsolved issues. Semin Cell Dev Biol. 2021;111:32-39. doi: 10.1016/j.semcdb.2020.05.013
- Takebe T, Sekine K, Enomura M, et al. Vascularized and functional human liver from an iPSC-derived organ bud transplant. Nature. 2013;499(7459):481-484. doi: 10.1038/nature12271
- Watson CL, Mahe MM, Munera J, et al. An in vivo model of human small intestine using pluripotent stem cells. Nat Med. 2014;20(11):1310-1314. doi: 10.1038/nm.3737
- Van den Berg CW, Ritsma L, Avramut MC, et al. Renal subcapsular transplantation of PSC-derived kidney organoids induces neo-vasculogenesis and significant glomerular and tubular maturation in vivo. Stem Cell Reports. 2018;10(3):751-765. doi: 10.1016/j.stemcr.2018.01.041
- Dye BR, Dedhia PH, Miller AJ, et al. A bioengineered niche promotes in vivo engraftment and maturation of pluripotent stem cell derived human lung organoids. Elife. 2016;5:e19732. doi: 10.7554/eLife.19732
- Shi Y, Sun L, Wang M, et al. Vascularized human cortical organoids (vOrganoids) model cortical development in vivo. PLoS Biol. 2020;18(5):e3000705. doi: 10.1371/journal.pbio.3000705
- Worsdorfer P, Dalda N, Kern A, et al. Generation of complex human organoid models including vascular networks by incorporation of mesodermal progenitor cells. Sci Rep. 2019;9(1):15663. doi: 10.1038/s41598-019-52204-7
- Logan S, Arzua T, Canfield SG, et al. Studying human neurological disorders using induced pluripotent stem cells: From 2D monolayer to 3D organoid and blood brain barrier models. Compr Physiol. 2019;9(2):565-611. doi: 10.1002/cphy.c180025
- Idris F, Hanna Muharram S, Zaini Z, Diah S. Establishment of murine in vitro blood-brain barrier models using immortalized cell lines: Co-cultures of brain endothelial cells, astrocytes, and neurons. bioRxiv. 2018:435990. doi: 10.1101/435990
- Wang Y, Wang N, Cai B, Wang GY, Li J, Piao XX. In vitro model of the blood-brain barrier established by co-culture of primary cerebral microvascular endothelial and astrocyte cells. Neural Regen Res. 2015;10(12):2011-2017. doi: 10.4103/1673-5374.172320
- Al Ahmad A, Taboada CB, Gassmann M, Ogunshola OO. Astrocytes and pericytes differentially modulate blood-brain barrier characteristics during development and hypoxic insult. J Cereb Blood Flow Metab. 2011;31(2):693-705. doi: 10.1038/jcbfm.2010.148
- Ham O, Jin YB, Kim J, Lee MO. Blood vessel formation in cerebral organoids formed from human embryonic stem cells. Biochem Biophys Res Commun. 2020;521(1):84-90. doi: 10.1016/j.bbrc.2019.10.079
- Shaji M, Tamada A, Fujimoto K, Muguruma K, Karsten SL, Yokokawa R. Deciphering potential vascularization factors of on-chip co-cultured hiPSC-derived cerebral organoids. Lab Chip. 2024;24(4):680-696. doi: 10.1039/d3lc00930k
- Ream MW, Randolph LN, Jiang Y, Chang Y, Bao X, Lian XL. Direct programming of human pluripotent stem cells into endothelial progenitors with SOX17 and FGF2. Stem Cell Reports. 2024;19(4):579-595. doi: 10.1016/j.stemcr.2024.02.006
- Lian X, Bao X, Al-Ahmad A, et al. Efficient differentiation of human pluripotent stem cells to endothelial progenitors via small-molecule activation of WNT signaling. Stem Cell Reports. 2014;3(5):804-816. doi: 10.1016/j.stemcr.2014.09.005
- Song L, Yuan X, Jones Z, et al. Assembly of human stem cell-derived cortical spheroids and vascular spheroids to model 3-d brain-like tissues. Sci Rep. 2019;9(1):5977. doi: 10.1038/s41598-019-42439-9
- Ahn Y, An JH, Yang HJ, et al. Human blood vessel organoids penetrate human cerebral organoids and form a vessel-like system. Cells. 2021;10(8):2036. doi: 10.3390/cells10082036
- Aisenbrey EA, Murphy WL. Synthetic alternatives to matrigel. Nat Rev Mater. 2020;5(7):539-551. doi: 10.1038/s41578-020-0199-8
- Kim YH, Choi SH, D’Avanzo C, et al. A 3D human neural cell culture system for modeling Alzheimer’s disease. Nat Protoc. 2015;10(7):985-1006. doi: 10.1038/nprot.2015.065
- Park J, Wetzel I, Marriott I, et al. A 3D human triculture system modeling neurodegeneration and neuroinflammation in Alzheimer’s disease. Nat Neurosci. 2018;21(7):941-951. doi: 10.1038/s41593-018-0175-4
- Kroll KT, Homan KA, Uzel SGM, et al. A perfusable, vascularized kidney organoid-on-chip model. Biofabrication. 2024;16(4): 045003. doi: 10.1088/1758-5090/ad5ac0
- Quintard C, Tubbs E, Jonsson G, et al. A microfluidic platform integrating functional vascularized organoids-on-chip. Nat Commun. 2024;15(1):1452. doi: 10.1038/s41467-024-45710-4
- Tan SY, Feng X, Cheng LKW, Wu AR. Vascularized human brain organoid on-chip. Lab Chip. 2023;23(12):2693-2709. doi: 10.1039/d2lc01109c
- LaMontagne E, Muotri AR, Engler AJ. Recent advancements and future requirements in vascularization of cortical organoids. Front Bioeng Biotechnol. 2022;10:1048731. doi: 10.3389/fbioe.2022.1048731
- Saldin LT, Cramer MC, Velankar SS, White LJ, Badylak SF. Extracellular matrix hydrogels from decellularized tissues: Structure and function. Acta Biomater. 2017;49:1-15. doi: 10.1016/j.actbio.2016.11.068
- Zhang Y, He Y, Bharadwaj S, et al. Tissue-specific extracellular matrix coatings for the promotion of cell proliferation and maintenance of cell phenotype. Biomaterials. 2009;30(23- 24):4021-4028. doi: 10.1016/j.biomaterials.2009.04.005
- DeQuach JA, Yuan SH, Goldstein LS, Christman KL. Decellularized porcine brain matrix for cell culture and tissue engineering scaffolds. Tissue Eng Part A. 2011;17(21- 22):2583-2592. doi: 10.1089/ten.TEA.2010.0724
- Simsa R, Rothenbucher T, Gurbuz H, et al. Brain organoid formation on decellularized porcine brain ECM hydrogels. PLoS One. 2021;16(1):e0245685. doi: 10.1371/journal.pone.0245685
- Sood D, Chwalek K, Stuntz E, et al. Fetal brain extracellular matrix boosts neuronal network formation in 3D bioengineered model of cortical brain tissue. ACS Biomater Sci Eng. 2016;2(1):131-140. doi: 10.1021/acsbiomaterials.5b00446
- Jin Y, Lee JS, Kim J, et al. Three-dimensional brain-like microenvironments facilitate the direct reprogramming of fibroblasts into therapeutic neurons. Nat Biomed Eng. 2018;2(7):522-539. doi: 10.1038/s41551-018-0260-8
- Yi HG, Jeong YH, Kim Y, et al. A bioprinted human-glioblastoma-on-a-chip for the identification of patient-specific responses to chemoradiotherapy. Nat Biomed Eng. 2019;3(7):509-519. doi: 10.1038/s41551-019-0363-x
- Koh I, Cha J, Park J, Choi J, Kang SG, Kim P. The mode and dynamics of glioblastoma cell invasion into a decellularized tissue-derived extracellular matrix-based three-dimensional tumor model. Sci Rep. 2018;8(1):4608. doi: 10.1038/s41598-018-22681-3
- Tan J, Zhang QY, Song YT, et al. Accelerated bone defect regeneration through sequential activation of the M1 and M2 phenotypes of macrophages by a composite BMP-2@ SIS hydrogel: An immunomodulatory perspective. Compos B Eng. 2022;243:110149. doi: 10.1016/j.compositesb.2022.110149
- Nie R, Zhang QY, Tan J, et al. EGCG modified small intestine submucosa promotes wound healing through immunomodulation. Compos B Eng. 2023;267:111005. doi: 10.1016/j.compositesb.2023.111005
- Heo JH, Kang D, Seo SJ, Jin Y. Engineering the extracellular matrix for organoid culture. Int J Stem Cells. 2022;15(1):60-69. doi: 10.15283/ijsc21190
- Garreta E, Moya-Rull D, Marco A, et al. Natural hydrogels support kidney organoid generation and promote in vitro angiogenesis. Adv Mater. 2024;36(34):e2400306. doi: 10.1002/adma.202400306
- Kim J, Kim J, Gao G, et al. Bioprinted organoids platform with tumor vasculature for implementing precision personalized medicine targeted towards gastric cancer. Adv Funct Mater. 2024;34(11):2306676. doi: 10.1002/adfm.202306676
- Katt ME, Linville RM, Mayo LN, Xu ZS, Searson PC. Functional brain-specific microvessels from iPSC-derived human brain microvascular endothelial cells: The role of matrix composition on monolayer formation. Fluids Barriers CNS. 2018;15(1):7. doi: 10.1186/s12987-018-0092-7
- Gifre-Renom L, Daems M, Luttun A, Jones EAV. Organ-specific endothelial cell differentiation and impact of microenvironmental cues on endothelial heterogeneity. Int J Mol Sci. 2022;23(3):1477. doi: 10.3390/ijms23031477
- Murphy AR, Laslett A, O’Brien CM, Cameron NR. Scaffolds for 3D in vitro culture of neural lineage cells. Acta Biomater. 2017;54:1-20. doi: 10.1016/j.actbio.2017.02.046
- Bourke JL, Quigley AF, Duchi S, et al. Three‐dimensional neural cultures produce networks that mimic native brain activity. J Tissue Eng Regen Med. 2018;12(2):490-493. doi: 10.1002/term.2508
- Iwashita M, Ohta H, Fujisawa T, et al. Brain-stiffness-mimicking tilapia collagen gel promotes the induction of dorsal cortical neurons from human pluripotent stem cells. Sci Rep. 2019;9(1):3068. doi: 10.1038/s41598-018-38395-5
- Zafeiriou MP, Bao G, Hudson J, et al. Developmental GABA polarity switch and neuronal plasticity in bioengineered neuronal organoids. Nat Commun. 2020;11(1):3791. doi: 10.1038/s41467-020-17521-w
- Sood D, Tang-Schomer M, Pouli D, et al. 3D extracellular matrix microenvironment in bioengineered tissue models of primary pediatric and adult brain tumors. Nat Commun. 2019;10(1):4529. doi: 10.1038/s41467-019-12420-1
- Shin Y, Choi SH, Kim E, et al. Blood-brain barrier dysfunction in a 3D in vitro model of Alzheimer’s disease. Adv Sci (Weinh). 2019;6(20):1900962. doi: 10.1002/advs.201900962
- Broguiere N, Isenmann L, Zenobi-Wong M. Novel enzymatically cross-linked hyaluronan hydrogels support the formation of 3D neuronal networks. Biomaterials. 2016;99:47-55. doi: 10.1016/j.biomaterials.2016.04.036
- Zhang ZN, Freitas BC, Qian H, et al. Layered hydrogels accelerate iPSC-derived neuronal maturation and reveal migration defects caused by MeCP2 dysfunction. Proc Natl Acad Sci U S A. 2016;113(12):3185-3190. doi: 10.1073/pnas.1521255113
- Silva LP, Pirraco RP, Santos TC, et al. Neovascularization induced by the hyaluronic acid-based spongy-like hydrogels degradation products. ACS Appl Mater Interfaces. 2016;8(49):33464-33474. doi: 10.1021/acsami.6b11684
- Hagbard L, Cameron K, August P, et al. Developing defined substrates for stem cell culture and differentiation. Philos Trans R Soc Lond B Biol Sci. 2018;373(1750):20170230. doi: 10.1098/rstb.2017.0230
- Alcaide D, Alric B, Cacheux J, et al. Laminin and hyaluronan supplementation of collagen hydrogels enhances endothelial function and tight junction expression on three-dimensional cylindrical microvessel-on-a-chip. Biochem Biophys Res Commun. 2024;724:150234. doi: 10.1016/j.bbrc.2024.150234
- Stamati K, Priestley JV, Mudera V, Cheema U. Laminin promotes vascular network formation in 3D in vitro collagen scaffolds by regulating VEGF uptake. Exp Cell Res. 2014;327(1):68-77. doi: 10.1016/j.yexcr.2014.05.012
- Gan Z, Qin X, Liu H, Liu J, Qin J. Recent advances in defined hydrogels in organoid research. Bioact Mater. 2023;28:386-401. doi: 10.1016/j.bioactmat.2023.06.004
- Papadimitriou C, Celikkaya H, Cosacak MI, et al. 3D culture method for Alzheimer’s disease modeling reveals interleukin-4 rescues Aβ42-induced loss of human neural stem cell plasticity. Dev Cell. 2018;46(1):85-101.e8. doi: 10.1016/j.devcel.2018.06.005
- Schwartz MP, Hou Z, Propson NE, et al. Human pluripotent stem cell-derived neural constructs for predicting neural toxicity. Proc Natl Acad Sci U S A. 2015;112(40):12516-12521. doi: 10.1073/pnas.1516645112
- Jiang L, Shen Y, Liu Y, Zhang L, Jiang W. Making human pancreatic islet organoids: Progresses on the cell origins, biomaterials and three-dimensional technologies. Theranostics. 2022;12(4):1537-1556. doi: 10.7150/thno.66670
- Jang H, Kim SH, Koh Y, Yoon KJ. Engineering brain organoids: Toward mature neural circuitry with an intact cytoarchitecture. Int J Stem Cells. 2022;15(1):41-59. doi: 10.15283/ijsc22004
- Marchini A, Favoino C, Gelain F. Multi-functionalized self-assembling peptides as reproducible 3D cell culture systems enabling differentiation and survival of various human neural stem cell lines. Front Neurosci. 2020;14:413. doi: 10.3389/fnins.2020.00413
- Zhang S, Wan Z, Kamm RD. Vascularized organoids on a chip: Strategies for engineering organoids with functional vasculature. Lab Chip. 2021;21(3):473-488. doi: 10.1039/d0lc01186j
- Zhao X, Xu Z, Xiao L, et al. Review on the vascularization of organoids and organoids-on-a-C hip. Front Bioeng Biotechnol. 2021;9:637048. doi: 10.3389/fbioe.2021.637048
- Adriani G, Ma D, Pavesi A, Kamm RD, Goh EL. A 3D neurovascular microfluidic model consisting of neurons, astrocytes and cerebral endothelial cells as a blood-brain barrier. Lab Chip. 2017;17(3):448-459. doi: 10.1039/c6lc00638h
- Osaki T, Sivathanu V, Kamm RD. Engineered 3D vascular and neuronal networks in a microfluidic platform. Sci Rep. 2018;8(1):5168. doi: 10.1038/s41598-018-23512-1
- Gong J, Gong Y, Zou T, et al. A controllable perfusion microfluidic chip for facilitating the development of retinal ganglion cells in human retinal organoids. Lab Chip. 2023;23(17):3820-3836. doi: 10.1039/d3lc00054k
- Abdulla A, Chen S, Chen Z, et al. Three-dimensional microfluidics with dynamic fluidic perturbation promotes viability and uniformity of human cerebral organoids. Biosens Bioelectron. 2023;240:115635. doi: 10.1016/j.bios.2023.115635
- Seiler ST, Mantalas GL, Selberg J, et al. Modular automated microfluidic cell culture platform reduces glycolytic stress in cerebral cortex organoids. Sci Rep. 2022;12(1):20173. doi: 10.1038/s41598-022-20096-9
- Salmon I, Grebenyuk S, Abdel Fattah AR, et al. Engineering neurovascular organoids with 3D printed microfluidic chips. Lab Chip. 2022;22(8):1615-1629. doi: 10.1039/d1lc00535a
- Osaki T, Uzel SGM, Kamm RD. Microphysiological 3D model of amyotrophic lateral sclerosis (ALS) from human iPS-derived muscle cells and optogenetic motor neurons. Sci Adv. 2018;4(10):eaat5847. doi: 10.1126/sciadv.aat5847
- Maoz BM, Herland A, FitzGerald EA, et al. A linked organ-on-chip model of the human neurovascular unit reveals the metabolic coupling of endothelial and neuronal cells. Nat Biotechnol. 2018;36(9):865-874. doi: 10.1038/nbt.4226
- Grebenyuk S, Abdel Fattah AR, Kumar M, et al. Large-scale perfused tissues via synthetic 3D soft microfluidics. Nat Commun. 2023;14(1):193. doi: 10.1038/s41467-022-35619-1
- Kajtez J, Nilsson F, Fiorenzano A, Parmar M, Emneus J. 3D biomaterial models of human brain disease. Neurochem Int 2021;147:105043. doi: 10.1016/j.neuint.2021.105043
- Miny L, Maisonneuve BGC, Quadrio I, Honegger T. Modeling neurodegenerative diseases using in vitro compartmentalized microfluidic devices. Front Bioeng Biotechnol. 2022;10:919646. doi: 10.3389/fbioe.2022.919646
- Amirifar L, Shamloo A, Nasiri R, et al. Brain-on-a-chip: Recent advances in design and techniques for microfluidic models of the brain in health and disease. Biomaterials. 2022;285:121531. doi: 10.1016/j.biomaterials.2022.121531
- Richards D, Jia J, Yost M, Markwald R, Mei Y. 3D bioprinting for vascularized tissue fabrication. Ann Biomed Eng. 2017;45(1):132-147. doi: 10.1007/s10439-016-1653-z
- Lin TY, Do T, Kwon P, Lillehoj PB. 3D printed metal molds for hot embossing plastic microfluidic devices. Lab Chip. 2017;17(2):241-247. doi: 10.1039/c6lc01430e
- Gomez K, Yarmey VR, Mane H, San-Miguel A. Microfluidic and computational tools for neurodegeneration studies. Annu Rev Chem Biomol Eng. 2025;16. doi: 10.1146/annurev-chembioeng-082223-054547
- Ko J, Park D, Lee J, et al. Microfluidic high-throughput 3D cell culture. Nat Rev Bioeng. 2024;2(6):453-469. doi: 10.1038/s44222-024-00163-8
- Syama S, Mohanan P. Microfluidic based human-on-a-chip: A revolutionary technology in scientific research. Trends Food Sci Technol. 2021;110:711-728. doi: 10.1016/j.tifs.2021.02.049
- Chen C, Rengarajan V, Kjar A, Huang Y. A matrigel-free method to generate matured human cerebral organoids using 3D-printed microwell arrays. Bioact Mater. 2021;6(4):1130-1139. doi: 10.1016/j.bioactmat.2020.10.003
- Beduer A, Braschler T, Peric O, et al. A compressible scaffold for minimally invasive delivery of large intact neuronal networks. Adv Healthc Mater. 2015;4(2):301-312. doi: 10.1002/adhm.201400250
- Jurga M, Dainiak MB, Sarnowska A, et al. The performance of laminin-containing cryogel scaffolds in neural tissue regeneration. Biomaterials. 2011;32(13):3423-3434. doi: 10.1016/j.biomaterials.2011.01.049
- Rothenbucher TSP, Gurbuz H, Pereira MP, Heiskanen A, Emneus J, Martinez-Serrano A. Next generation human brain models: Engineered flat brain organoids featuring gyrification. Biofabrication. 2021;13(1):011001. doi: 10.1088/1758-5090/abc95e
- Xu L, Ding H, Wu S, et al. Artificial meshed vessel-induced dimensional breaking growth of human brain organoids and multiregional assembloids. ACS Nano. 2024;18(38):26201-26014. doi: 10.1021/acsnano.4c07844
- Ren Y, Yang X, Ma Z, et al. Developments and opportunities for 3D bioprinted organoids. Int J Bioprint. 2021;7(3):364. doi: 10.18063/ijb.v7i3.364
- Sukhinich K, Shakirova K, Dashinimaev E, Aleksandrova M. Development of 3D cerebral aggregates in the brain ventricles of adult mice. Russ J Dev Biol. 2021;52(3):164-175. doi: 10.1134/S1062360421030061
- Daviaud N, Friedel RH, Zou H. Vascularization and engraftment of transplanted human cerebral organoids in mouse cortex. eNeuro. 2018;5(6). doi: 10.1523/ENEURO.0219-18.2018
- Mansour AA, Gonçalves JT, Bloyd CW, et al. An in vivo model of functional and vascularized human brain organoids. Nat Biotechnol. 2018;36(5):432-441. doi: 10.1038/nbt.4127
- Revah O, Gore F, Kelley KW, et al. Maturation and circuit integration of transplanted human cortical organoids. Nature. 2022;610(7931):319-326. doi: 10.1038/s41586-022-05277-w
- Chiaradia I, Lancaster MA. Brain organoids for the study of human neurobiology at the interface of in vitro and in vivo. Nat Neurosci. 2020;23(12):1496-1508. doi: 10.1038/s41593-020-00730-3
- Pasca SP, Arlotta P, Bateup HS, et al. A framework for neural organoids, assembloids and transplantation studies. Nature. 2025;639(8054):315-320. doi: 10.1038/s41586-024-08487-6
- Alameri A. Microfluidic-based Organoid Vascularization in PDMS Devices Replicated from 3D Printed Molds. Canada: McGill University; 2022.
- Xu C, Alameri A, Leong W, et al. Multiscale engineering of brain organoids for disease modeling. Adv Drug Deliv Rev. 2024;210:115344. doi: 10.1016/j.addr.2024.115344
- Bhalerao A, Sivandzade F, Archie SR, Chowdhury EA, Noorani B, Cucullo L. In vitro modeling of the neurovascular unit: Advances in the field. Fluids Barriers CNS. 2020;17(1):22. doi: 10.1186/s12987-020-00183-7
- D’Antoni C, Mautone L, Sanchini C, et al. Unlocking neural function with 3D in vitro models: A technical review of self-assembled, guided, and bioprinted brain organoids and their applications in the study of neurodevelopmental and neurodegenerative disorders. Int J Mol Sci. 2023;24(13):10762. doi: 10.3390/ijms241310762
- Depla JA, Mulder LA, de Sá RV, et al. Human brain organoids as models for central nervous system viral infection. Viruses. 2022;14(3):634. doi: 10.3390/v14030634
- Fan W, Christian KM, Song H, Ming GL. Applications of brain organoids for infectious diseases. J Mol Biol. 2022;434(3):167243. doi: 10.1016/j.jmb.2021.167243
- Sato Y, Asahi T, Kataoka K. Integrative single-cell RNA-seq analysis of vascularized cerebral organoids. BMC Biol. 2023;21(1):245. doi: 10.1186/s12915-023-01711-1
- Chai YC, To SK, Simorgh S, et al. Spatially self‐organized three‐dimensional neural concentroid as a novel reductionist humanized model to study neurovascular development. Adv Sci. 2024;11(5):2304421. doi: 10.1002/advs.202304421
- Kumarasamy M, Sosnik A. Heterocellular spheroids of the neurovascular blood-brain barrier as a platform for personalized nanoneuromedicine. Iscience. 2021;24(3):102183. doi: 10.1016/j.isci.2021.102183
- Abijo AZ, Olatunji SY, Adelodun ST, Asamu MO, Omeiza NA. Modeling Alzheimer’s disease using cerebral organoids: Current challenges and prospects. Brain Organoid Syst Neurosci J. 2024;2:53-63. doi: 10.1016/j.bosn.2024.09.001
- Kumarasamy M, Sosnik A. Multicellular organoids of the neurovascular blood-brain barrier: A new platform for precision neuronanomedicine. bioRxiv. 2020:249326. doi: 10.1101/2020.08.14.249326
- Urrestizala-Arenaza N, Cerchio S, Cavaliere F, Magliaro C. Limitations of human brain organoids to study neurodegenerative diseases: A manual to survive. Front Cell Neurosci. 2024;18:1419526. doi: 10.3389/fncel.2024.1419526
- Balikov DA, Neal EH, Lippmann ES. Organotypic neurovascular models: Past results and future directions. Trends Mol Med. 2020;26(3):273-284. doi: 10.1016/j.molmed.2019.09.010
- Mulay AR, Hwang J, Kim DH. Microphysiological blood-brain barrier systems for disease modeling and drug development. Adv Healthc Mater. 2024;13(21):e2303180. doi: 10.1002/adhm.202303180
- Hussain B, Fang C, Chang J. Blood-brain barrier breakdown: An emerging biomarker of cognitive impairment in normal aging and dementia. Front Neurosci. 2021;15:688090. doi: 10.3389/fnins.2021.688090
- Ko EC, Spitz S, Pramotton FM, et al. Accelerating the in vitro emulation of Alzheimer’s disease-associated phenotypes using a novel 3D blood-brain barrier neurosphere co-culture model. Front Bioeng Biotechnol. 2023;11:1251195. doi: 10.3389/fbioe.2023.1251195
- Song G, Zhao M, Chen H, et al. The application of brain organoid technology in stroke research: Challenges and prospects. Front Cell Neurosci. 2021;15:646921. doi: 10.3389/fncel.2021.646921
- Nzou G, Wicks RT, VanOstrand NR, et al. Multicellular 3D neurovascular unit model for assessing hypoxia and neuroinflammation induced blood-brain barrier dysfunction. Sci Rep. 2020;10(1):9766. doi: 10.1038/s41598-020-66487-8
- Grebenyuk S, Ranga A. Engineering organoid vascularization. Front Bioeng Biotechnol. 2019;7:39. doi: 10.3389/fbioe.2019.00039
- Mo F, Pellerino A, Soffietti R, Ruda R. Blood-brain barrier in brain tumors: Biology and clinical relevance. Int J Mol Sci. 2021;22(23):12654. doi: 10.3390/ijms222312654
- Bergmann S, Lawler SE, Qu Y, et al. Blood-brain-barrier organoids for investigating the permeability of CNS therapeutics. Nat Protoc. 2018;13(12):2827-2843. doi: 10.1038/s41596-018-0066-x
- Hong SJ, Bock M, Zhang S, An SB, Han I. Therapeutic transplantation of human central nervous system organoids for neural reconstruction. Int J Mol Sci. 2024;25(15):8540. doi: 10.3390/ijms25158540
- Wang SN, Wang Z, Xu TY, Cheng MH, Li WL, Miao CY. Cerebral organoids repair ischemic stroke brain injury. Transl Stroke Res. 2020;11(5):983-1000. doi: 10.1007/s12975-019-00773-0
- Cao SY, Tao MD, Lou SN, et al. Functional reconstruction of the impaired cortex and motor function by hMGEOs transplantation in stroke. Biochem Biophys Res Commun. 2023;671:87-95. doi: 10.1016/j.bbrc.2023.06.010
- Mulder LA, Depla JA, Sridhar A, Wolthers K, Pajkrt D, Vieira de Sa R. A beginner’s guide on the use of brain organoids for neuroscientists: A systematic review. Stem Cell Res Ther. 2023;14(1):87. doi: 10.1186/s13287-023-03302-x
- Lahimchi MR, Maroufi F, Maali A. Induced pluripotent stem cell-derived chimeric antigen receptor T cells: The intersection of stem cells and immunotherapy. Cell Reprogram. 2023;25(5):195-211. doi: 10.1089/cell.2023.0041
- Tahbaz M, Yoshihara E. Immune protection of stem cell-derived islet cell therapy for treating diabetes. Front Endocrinol (Lausanne). 2021;12:716625. doi: 10.3389/fendo.2021.716625
- Petrus-Reurer S, Romano M, Howlett S, Jones JL, Lombardi G, Saeb-Parsy K. Immunological considerations and challenges for regenerative cellular therapies. Commun Biol. 2021;4(1):798. doi: 10.1038/s42003-021-02237-4
- Gravina A, Tediashvili G, Zheng Y, et al. Synthetic immune checkpoint engagers protect HLA-deficient iPSCs and derivatives from innate immune cell cytotoxicity. Cell Stem Cell. 2023;30(11):1538-1548.e4. doi: 10.1016/j.stem.2023.10.003
- Urzì O, Gasparro R, Costanzo E, et al. Three-dimensional cell cultures: The bridge between in vitro and in vivo models. Int J Mol Sci. 2023;24(15):12046. doi: 10.3390/ijms241512046
- Yang G, Mahadik B, Choi JY, Fisher JP. Vascularization in tissue engineering: Fundamentals and state-of-art. Prog Biomed Eng (Bristol). 2020;2(1):012002. doi: 10.1088/2516-1091/ab5637
- Lancaster MA, Knoblich JA. Generation of cerebral organoids from human pluripotent stem cells. Nat Protoc. 2014;9(10):2329-2340. doi: 10.1038/nprot.2014.158
- Lou YR, Leung AW. Next generation organoids for biomedical research and applications. Biotechnol Adv. 2018;36(1):132-149. doi: 10.1016/j.biotechadv.2017.10.005
- Wimmer RA, Leopoldi A, Aichinger M, et al. Human blood vessel organoids as a model of diabetic vasculopathy. Nature. 2019;565(7740):505-510. doi: 10.1038/s41586-018-0858-8
- Marton RM, Pasca SP. Organoid and assembloid technologies for investigating cellular crosstalk in human brain development and disease. Trends Cell Biol. 2020;30(2):133-143. doi: 10.1016/j.tcb.2019.11.004
- Bhaduri A, Andrews MG, Mancia Leon W, et al. Cell stress in cortical organoids impairs molecular subtype specification. Nature. 2020;578(7793):142-148. doi: 10.1038/s41586-020-1962-0
- Workman MJ, Mahe MM, Trisno S, et al. Engineered human pluripotent-stem-cell-derived intestinal tissues with a functional enteric nervous system. Nat Med. 2017;23(1):49-59. doi: 10.1038/nm.4233
- Fernandes S, Revanna J, Pratt J, Hayes N, Marchetto MC, Gage FH. Modeling Alzheimer’s disease using human cell derived brain organoids and 3D models. Front Neurosci. 2024;18:1434945. doi: 10.3389/fnins.2024.1434945
- Koplin JJ, Savulescu J. Moral limits of brain organoid research. J Law Med Ethics. 2019;47(4):760-767. doi: 10.1177/1073110519897789
- Gao M, Zhu X, Yang G, Bao J, Bu H. CRISPR/Cas9-mediated gene editing in porcine models for medical research. DNA Cell Biol. 2021;40(12):1462-1475. doi: 10.1089/dna.2020.6474
- Ashok A, Choudhury D, Fang Y, Hunziker W. Towards manufacturing of human organoids. Biotechnol Adv. 2020;39:107460. doi: 10.1016/j.biotechadv.2019.107460
- Lancaster MA, Knoblich JA. Organogenesis in a dish: Modeling development and disease using organoid technologies. Science. 2014;345(6194):1247125. doi: 10.1126/science.1247125
- Xiao Y, Kim D, Dura B, et al. Ex vivo dynamics of human glioblastoma cells in a microvasculature-on-a-chip system correlates with tumor heterogeneity and subtypes. Adv Sci (Weinh). 2019;6(8):1801531. doi: 10.1002/advs.201801531
- Adams RH, Alitalo K. Molecular regulation of angiogenesis and lymphangiogenesis. Nat Rev Mol Cell Biol. 2007;8(6):464-478. doi: 10.1038/nrm2183
- Kook MG, Lee SE, Shin N, et al. Generation of cortical brain organoid with vascularization by assembling with vascular spheroid. Int J Stem Cells. 2022;15(1):85-94. doi: 10.15283/ijsc21157
- Werschler N, Quintard C, Nguyen S, Penninger J. Engineering next generation vascularized organoids. Atherosclerosis. 2024;398:118529. doi: 10.1016/j.atherosclerosis.2024.118529
- Nwokoye PN, Abilez OJ. Bioengineering methods for vascularizing organoids. Cell Rep Methods. 2024;4(6):100779. doi: 10.1016/j.crmeth.2024.100779
- Sweeney MD, Ayyadurai S, Zlokovic BV. Pericytes of the neurovascular unit: Key functions and signaling pathways. Nat Neurosci. 2016;19(6):771-783. doi: 10.1038/nn.4288
- Zhang Y, Yang X. The roles of TGF-β signaling in cerebrovascular diseases. Front Cell Dev Biol. 2020;8:567682. doi: 10.3389/fcell.2020.567682
- Wang X, Bijonowski BM, Kurniawan NA. Vascularizing organoids to promote long-term organogenesis on a chip. Organoids. 2023;2(4):239-255. doi: 10.3390/organoids2040019
- Amini A, Masoumi Moghaddam S, Morris DL, Pourgholami MH. The critical role of vascular endothelial growth factor in tumor angiogenesis. Curr Cancer Drug Targets. 2012;12(1):23-43. doi: 10.2174/156800912798888956
- Liu Y, Li F, Yang YT, et al. IGFBP2 promotes vasculogenic mimicry formation via regulating CD144 and MMP2 expression in glioma. Oncogene. 2019;38(11):1815-1831. doi: 10.1038/s41388-018-0525-4
- Zegarra-Valdivia JA, Pignatelli J, Nunez A, Torres Aleman I. The role of insulin-like growth factor I in mechanisms of resilience and vulnerability to sporadic Alzheimer’s disease. Int J Mol Sci. 2023;24(22):16440. doi: 10.3390/ijms242216440
- Ricard N, Ciais D, Levet S, et al. BMP9 and BMP10 are critical for postnatal retinal vascular remodeling. Blood. 2012;119(25):6162-6171. doi: 10.1182/blood-2012-01-407593