Brain organoids: An innovative model for central nervous system diseases in neurosurgery
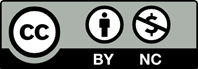
Central nervous system (CNS) plays an important role in regulating sensory perception, cognition, motor control, and homeostasis. However, these functions can be impaired by diseases such as traumatic brain injury (TBI), glioblastoma multiforme (GBM), and Parkinson’s disease (PD), thereby reducing life quality. Traditional experimental models fall short in capturing CNS pathology. Brain organoids (BOs), derived from human induced pluripotent stem cells and human embryonic stem cells, replicate human brain structure and function, offering new insights into CNS diseases. TBI-induced neural death and neuroinflammation can be modeled using BOs but further refinement is required to mirror complex brain architecture. For GBM, BOs provide insights into the tumor microenvironment and glioblastoma stem cells behavior, aiding drug screening. In PD research, BOs replicate dopaminergic neuron loss, but long-term stability and reproducibility remain challenging. While BOs offer improved insight into CNS pathology, key challenges remain, including the need for better simulation of brain complexity, long-term stability, and reproducibility. In this review, we focus on the application of BOs in modeling neurological diseases related to neurosurgical practice. We also discuss their application scenarios, construction strategies, and clinical relevance, aiming to provide a reference for further research on organoid-based models, addressing the limitations of traditional models.
- Schuster M, Braun FK, Chiang DM, et al. Extracellular vesicles secreted by 3D tumor organoids are enriched for immune regulatory signaling biomolecules compared to conventional 2D glioblastoma cell systems. Front Immunol. 2024;15:1388769. doi: 10.3389/fimmu.2024.1388769
- He W, Kuang Y, Xing X, et al. Proteomic comparison of 3D and 2D glioma models reveals increased HLA-E expression in 3D models is associated with resistance to NK cell-mediated cytotoxicity. J Proteome Res. 2014;13(5):2272-2281. doi: 10.1021/pr500064m
- Dovonou A, Bolduc C, Soto Linan V, Gora C, Peralta Iii MR, Lévesque M. Animal models of Parkinson’s disease: Bridging the gap between disease hallmarks and research questions. Transl Neurodegener. 2023;12(1):36. doi: 10.1186/s40035-023-00368-8
- Hammond SM, Abendroth F, Goli L, et al. Antibody-oligonucleotide conjugate achieves CNS delivery in animal models for spinal muscular atrophy. JCI Insight. 2022;7(24):e154142. doi: 10.1172/jci.insight.154142
- Ayers KN, Carey SN, Lukacher AE. Understanding polyomavirus CNS disease - a perspective from mouse models. FEBS J. 2022;289(19):5744-5761. doi: 10.1111/febs.16083
- Zhu W, Xu L, Li X, Hu H, Lou S, Liu Y. iPSCs-derived neurons and brain organoids from patients. Handb Exp Pharmacol. 2023;281:59-81. doi: 10.1007/164_2023_657
- Schafer ST, Mansour AA, Schlachetzki JCM, et al. An in vivo neuroimmune organoid model to study human microglia phenotypes. Cell. 2023;186(10):2111-2126.e20. doi: 10.1016/j.cell.2023.04.022
- Shafique S. Stem cell-based region-specific brain organoids: Novel models to understand neurodevelopmental defects. Birth Defects Res. 2022;114(16):1003-1013. doi: 10.1002/bdr2.2004
- Lacalle-Aurioles M, Cassel de Camps C, Zorca CE, Beitel LK, Durcan TM. Applying hiPSCs and biomaterials towards an understanding and treatment of traumatic brain injury. Front Cell Neurosci. 2020;14:594304. doi: 10.3389/fncel.2020.594304
- Pazzin DB, Previato TTR, Budelon Gonçalves JI, et al. Induced pluripotent stem cells and organoids in advancing neuropathology research and therapies. Cells. 2024;13(9):745. doi: 10.3390/cells13090745
- Jgamadze D, Johnson VE, Wolf JA, et al. Modeling traumatic brain injury with human brain organoids. Curr Opin Biomed Eng. 2020;14:52-58. doi: 10.1016/j.cobme.2020.05.004
- Bellotti C, Samudyata S, Thams S, Sellgren CM, Rostami E. Organoids and chimeras: The hopeful fusion transforming traumatic brain injury research. Acta Neuropathol Commun. 2024;12(1):141. doi: 10.1186/s40478-024-01845-5
- Kim MS, Kim DH, Kang HK, Kook MG, Choi SW, Kang KS. Modeling of hypoxic brain injury through 3D human neural organoids. Cells. 2021;10(2):234. doi: 10.3390/cells10020234
- Hwang JW, Loisel-Duwattez J, Desterke C, et al. A novel neuronal organoid model mimicking glioblastoma (GBM) features from induced pluripotent stem cells (iPSC). Biochim Biophys Acta Gen Subj. 2020;1864(4):129540. doi: 10.1016/j.bbagen.2020.129540
- Duan J, Wang Y. Modeling nervous system tumors with human stem cells and organoids. Cell Regen. 2023;12(1):4. doi: 10.1186/s13619-022-00150-7
- Pawlowski KD, Duffy JT, Babak MV, Balyasnikova IV. Modeling glioblastoma complexity with organoids for personalized treatments. Trends Mol Med. 2023;29(4):282-296. doi: 10.1016/j.molmed.2023.01.002
- Sabate-Soler S, Nickels SL, Saraiva C, et al. Microglia integration into human midbrain organoids leads to increased neuronal maturation and functionality. Glia. 2022;70(7):1267-1288. doi: 10.1002/glia.24167
- Huang WK, Wong SZH, Pather SR, et al. Generation of hypothalamic arcuate organoids from human induced pluripotent stem cells. Cell Stem Cell. 2021;28(9):1657-1670.e10. doi: 10.1016/j.stem.2021.04.006
- Pasko VI, Churkina AS, Shakhov AS, Kotlobay AA, Alieva IB. Modeling of neurodegenerative diseases: ‘Step by Step’ and ‘Network’ organization of the complexes of model systems. Int J Mol Sci. 2022;24(1):604. doi: 10.3390/ijms24010604
- Karmirian K, Holubiec M, Goto-Silva L, et al. Modeling Alzheimer’s disease using human brain organoids. Methods Mol Biol. 2023;2561:135-158. doi: 10.1007/978-1-0716-2655-9_7
- Lee CT, Bendriem RM, Wu WW, Shen RF. 3D brain organoids derived from pluripotent stem cells: Promising experimental models for brain development and neurodegenerative disorders. J Biomed Sci. 2017;24(1):59. doi: 10.1186/s12929-017-0362-8
- Takahashi K, Tanabe K, Ohnuki M, et al. Induction of pluripotent stem cells from adult human fibroblasts by defined factors. Cell. 2007;131(5):861-872. doi: 10.1016/j.cell.2007.11.019
- Smith L, Quelch-Cliffe R, Liu F, Aguilar AH, Przyborski S. Evaluating strategies to assess the differentiation potential of human pluripotent stem cells: A review, analysis and call for innovation. Stem Cell Rev Rep. 2024;21:107-125. doi: 10.1007/s12015-024-10793-5
- Sidhaye J, Knoblich JA. Brain organoids: An ensemble of bioassays to investigate human neurodevelopment and disease. Cell Death Differ. 2021;28(1):52-67. doi: 10.1038/s41418-020-0566-4
- Logan S, Arzua T, Yan Y, et al. Dynamic characterization of structural, molecular, and electrophysiological phenotypes of human-induced pluripotent stem cell-derived cerebral organoids, and comparison with fetal and adult gene profiles. Cells. 2020;9(5):1301. doi: 10.3390/cells9051301
- Xiang Y, Tanaka Y, Patterson B, et al. Fusion of regionally specified hPSC-derived organoids models human brain development and interneuron migration. Cell Stem Cell. 2017;21(3):383-398.e7. doi: 10.1016/j.stem.2017.07.007
- Zhang DY, Song H, Ming GL. Modeling neurological disorders using brain organoids. Semin Cell Dev Biol. 2021;111:4-14. doi: 10.1016/j.semcdb.2020.05.026
- Ho BX, Pek NMQ, Soh BS. Disease modeling using 3D organoids derived from human induced pluripotent stem cells. Int J Mol Sci. 2018;19(4):936. doi: 10.3390/ijms19040936
- Velasco S, Kedaigle AJ, Simmons SK, et al. Individual brain organoids reproducibly form cell diversity of the human cerebral cortex. Nature. 2019;570(7762):523-527. doi: 10.1038/s41586-019-1289-x
- Deng W. Induced pluripotent stem cells: Paths to new medicines. A catalyst for disease modelling, drug discovery and regenerative therapy. EMBO Rep. 2010;11(3):161-165. doi: 10.1038/embor.2010.15
- Kim K, Doi A, Wen B, et al. Epigenetic memory in induced pluripotent stem cells. Nature. 2010;467(7313):285-290. doi: 10.1038/nature09342
- Qian X, Song H, Ming GL. Brain organoids: Advances, applications and challenges. Development. 2019;146(8):dev166074. doi: 10.1242/dev.166074
- Lokai T, Albin B, Qubbaj K, Tiwari AP, Adhikari P, Yang IH. A review on current brain organoid technologies from a biomedical engineering perspective. Exp Neurol. 2023;367:114461. doi: 10.1016/j.expneurol.2023.114461
- Fedorchak NJ, Iyer N, Ashton RS. Bioengineering tissue morphogenesis and function in human neural organoids. Semin Cell Dev Biol. 2021;111:52-59. doi: 10.1016/j.semcdb.2020.05.025
- Marti-Figueroa CR, Ashton RS. The case for applying tissue engineering methodologies to instruct human organoid morphogenesis. Acta Biomater. 2017;54:35-44. doi: 10.1016/j.actbio.2017.03.023
- Shah SB, Singh A. Cellular self-assembly and biomaterials-based organoid models of development and diseases. Acta Biomater. 2017;53:29-45. doi: 10.1016/j.actbio.2017.01.075
- Bose R, Banerjee S, Dunbar GL. Modeling neurological disorders in 3D organoids using human-derived pluripotent stem cells. Front Cell Dev Biol. 2021;9:640212. doi: 10.3389/fcell.2021.640212
- Cortes D, Pera MF. The genetic basis of inter-individual variation in recovery from traumatic brain injury. NPJ Regen Med. 2021;6(1):5. doi: 10.1038/s41536-020-00114-y
- Azzarelli R. Organoid models of glioblastoma to study brain tumor stem cells. Front Cell Dev Biol. 2020;8:220. doi: 10.3389/fcell.2020.00220
- Grenier K, Kao J, Diamandis P. Three-dimensional modeling of human neurodegeneration: Brain organoids coming of age. Mol Psychiatry. 2020;25(2):254-274. doi: 10.1038/s41380-019-0500-7
- Kim J, Koo BK, Knoblich JA. Human organoids: Model systems for human biology and medicine. Nat Rev Mol Cell Biol. 2020;21(10):571-584. doi: 10.1038/s41580-020-0259-3
- Lancaster MA, Corsini NS, Wolfinger S, et al. Guided self-organization and cortical plate formation in human brain organoids. Nat Biotechnol. 2017;35(7):659-666. doi: 10.1038/nbt.3906. Erratum in: Nat Biotechnol. 2018;36(10):1016. doi: 10.1038/nbt1018-1016a
- Gonzales-Aloy E, Ahmed-Cox A, Tsoli M, Ziegler DS, Kavallaris M. From cells to organoids: The evolution of blood-brain barrier technology for modelling drug delivery in brain cancer. Adv Drug Deliv Rev. 2023;196:114777. doi: 10.1016/j.addr.2023.114777
- Bergmann S, Lawler SE, Qu Y, et al. Blood-brain-barrier organoids for investigating the permeability of CNS therapeutics. Nat Protoc. 2018;13(12):2827-2843. doi: 10.1038/s41596-018-0066-x
- Driehuis E, Kretzschmar K, Clevers H. Establishment of patient-derived cancer organoids for drug-screening applications. Nat Protoc. 2020;15(10):3380-3409. doi: 10.1038/s41596-020-0379-4. Erratum in: Nat Protoc. 2021;16(12):5739. doi: 10.1038/s41596-021-00494-5
- Xu C, Yuan X, Hou P, Li Z, Wang C, Fang C, Tan Y. Development of glioblastoma organoids and their applications in personalized therapy. Cancer Biol Med. 2023;20(5):353-368. doi: 10.20892/j.issn.2095-3941.2023.0061
- Rybin MJ, Ivan ME, Ayad NG, Zeier Z. Organoid models of glioblastoma and their role in drug discovery. Front Cell Neurosci. 2021;15:605255. doi: 10.3389/fncel.2021.605255
- Fan W, Christian KM, Song H, Ming GL. Applications of brain organoids for infectious diseases. J Mol Biol. 2022;434(3):167243. doi: 10.1016/j.jmb.2021.167243
- Gabriel E, Ramani A, Altinisik N, Gopalakrishnan J. Human brain organoids to decode mechanisms of microcephaly. Front Cell Neurosci. 2020;14:115. doi: 10.3389/fncel.2020.00115
- Villanueva R. Advances in the knowledge and therapeutics of schizophrenia, major depression disorder, and bipolar disorder from human brain organoid research. Front Psychiatry. 2023;14:1178494. doi: 10.3389/fpsyt.2023.1178494
- Lancaster MA, Renner M, Martin CA, et al. Cerebral organoids model human brain development and microcephaly. Nature. 2013;501(7467):373-379. doi: 10.1038/nature12517
- Mellios N, Feldman DA, Sheridan SD, et al. MeCP2- regulated miRNAs control early human neurogenesis through differential effects on ERK and AKT signaling. Mol Psychiatry. 2018;23(4):1051-1065. doi: 10.1038/mp.2017.86
- Graham NSN, Jolly A, Zimmerman K, et al. Diffuse axonal injury predicts neurodegeneration after moderate-severe traumatic brain injury. Brain. 2020;143(12):3685-3698. doi: 10.1093/brain/awaa316
- Palmieri M, Frati A, Santoro A, Frati P, Fineschi V, Pesce A. Diffuse axonal injury: Clinical prognostic factors, molecular experimental models and the impact of the trauma related oxidative stress. An extensive review concerning milestones and advances. Int J Mol Sci. 2021;22(19):10865. doi: 10.3390/ijms221910865
- Graham NS, Sharp DJ. Understanding neurodegeneration after traumatic brain injury: From mechanisms to clinical trials in dementia. J Neurol Neurosurg Psychiatry. 2019;90(11):1221-1233. doi: 10.1136/jnnp-2017-317557
- Jha RM, Kochanek PM, Simard JM. Pathophysiology and treatment of cerebral edema in traumatic brain injury. Neuropharmacology. 2019;145(Pt B):230-246. doi: 10.1016/j.neuropharm.2018.08.004
- Ashina H, Porreca F, Anderson T, et al. Post-traumatic headache: Epidemiology and pathophysiological insights. Nat Rev Neurol. 2019;15(10):607-617. doi: 10.1038/s41582-019-0243-8
- Wang Q, Mergia E, Koesling D, Mittmann T. Nitric oxide/ cyclic guanosine monophosphate signaling via guanylyl cyclase isoform 1 mediates early changes in synaptic transmission and brain edema formation after traumatic brain injury. J Neurotrauma. 2021;38(12):1689-1701. doi: 10.1089/neu.2020.7364
- Zhang C, Liu C, Li F, et al. Extracellular mitochondria activate microglia and contribute to neuroinflammation in traumatic brain injury. Neurotox Res. 2022;40(6):2264-2277. doi: 10.1007/s12640-022-00566-8
- Robinson CP. Moderate and severe traumatic brain injury. Continuum (Minneap Minn). 2021;27(5):1278-1300. doi: 10.1212/CON.0000000000001036
- Al-Dahhak R, Khoury R, Qazi E, Grossberg GT. Traumatic brain injury, chronic traumatic encephalopathy, and Alzheimer disease. Clin Geriatr Med. 2018;34(4):617-635. doi: 10.1016/j.cger.2018.06.008
- Krishnamurthy K, Laskowitz DT. Cellular and molecular mechanisms of secondary neuronal injury following traumatic brain injury. In: Laskowitz D, Grant G, editors. Translational Research in Traumatic Brain Injury. Ch. 5. Boca Raton, FL: CRC Press/Taylor and Francis Group; 2016.
- Silvosa MJ, Mercado NR, Merlock N, et al. Understanding primary blast injury: High frequency pressure acutely disrupts neuronal network dynamics in cerebral organoids. J Neurotrauma. 2022;39(21-22):1575-1590. doi: 10.1089/neu.2022.0044
- Morrison B 3rd, Cater HL, Benham CD, Sundstrom LE. An in vitro model of traumatic brain injury utilising two-dimensional stretch of organotypic hippocampal slice cultures. J Neurosci Methods. 2006;150(2):192-201. doi: 10.1016/j.jneumeth.2005.06.014
- Ramirez S, Mukherjee A, Sepulveda S, et al. Modeling traumatic brain injury in human cerebral organoids. Cells. 2021;10(10):2683. doi: 10.3390/cells10102683
- Hamilton KA, Santhakumar V. Current ex vivo and in vitro approaches to uncovering mechanisms of neurological dysfunction after traumatic brain injury. Curr Opin Biomed Eng. 2020;14:18-24. doi: 10.1016/j.cobme.2020.05.001
- Tang-Schomer MD, White JD, Tien LW, et al. Bioengineered functional brain-like cortical tissue. Proc Natl Acad Sci U S A. 2014;111(38):13811-13816. doi: 10.1073/pnas.1324214111
- Chwalek K, Tang-Schomer MD, Omenetto FG, Kaplan DL. In vitro bioengineered model of cortical brain tissue. Nat Protoc. 2015;10(9):1362-1373. doi: 10.1038/nprot.2015.091
- Kadoshima T, Sakaguchi H, Nakano T, et al. Self-organization of axial polarity, inside-out layer pattern, and species-specific progenitor dynamics in human ES cell-derived neocortex. Proc Natl Acad Sci U S A. 2013;110(50):20284-20289. doi: 10.1073/pnas.1315710110
- Paşca AM, Sloan SA, Clarke LE, et al. Functional cortical neurons and astrocytes from human pluripotent stem cells in 3D culture. Nat Methods. 2015;12(7):671-678. doi: 10.1038/nmeth.3415
- Ramirez S, Mukherjee A, Sepulveda SE, et al. Protocol for controlled cortical impact in human cerebral organoids to model traumatic brain injury. STAR Protoc. 2021;2(4):100987. doi: 10.1016/j.xpro.2021.100987
- Brain injury awareness month - March 2019. MMWR Morb Mortal Wkly Rep. 2019;68(10):237. doi: 10.15585/mmwr.mm6810a1
- Petersilie L, Kafitz KW, Neu LA, et al. Protocol for the generation of cultured cortical brain organoid slices. STAR Protoc. 2024;5(3):103212. doi: 10.1016/j.xpro.2024.103212
- Petersilie L, Heiduschka S, Nelson JSE, et al. Cortical brain organoid slices (cBOS) for the study of human neural cells in minimal networks. iScience. 2024;27(4):109415. doi: 10.1016/j.isci.2024.109415
- Tang J, Zheng H, Feng Y, Zeng J, Ma S. Attachment culture of cortical organoids at the microwell air-liquid interface. STAR Protoc. 2023;4(3):102502. doi: 10.1016/j.xpro.2023.102502
- Qian X, Su Y, Adam CD, et al. Sliced human cortical organoids for modeling distinct cortical layer formation. Cell Stem Cell. 2020;26(5):766-781.e9. doi: 10.1016/j.stem.2020.02.002
- Hutchinson PJ, Kolias AG, Timofeev IS, et al. Trial of decompressive craniectomy for traumatic intracranial hypertension. N Engl J Med. 2016;375(12):1119-1130. doi: 10.1056/NEJMoa1605215
- Chukwurah E, Osmundsen A, Davis SW, Lizarraga SB. All together now: Modeling the interaction of neural with non-neural systems using organoid models. Front Neurosci. 2019;13:582. doi: 10.3389/fnins.2019.00582
- Raghupathi R, Graham DI, McIntosh TK. Apoptosis after traumatic brain injury. J Neurotrauma. 2000;17(10):927-938. doi: 10.1089/neu.2000.17.927
- Clark RS, Kochanek PM, Watkins SC, et al. Caspase-3 mediated neuronal death after traumatic brain injury in rats. J Neurochem. 2000;74(2):740-753. doi: 10.1046/j.1471-4159.2000.740740.x
- Lai JD, Berlind JE, Fricklas G, et al. KCNJ2 inhibition mitigates mechanical injury in a human brain organoid model of traumatic brain injury. Cell Stem Cell. 2024;31(4):519-536.e8. doi: 10.1016/j.stem.2024.03.004
- Beltrán SM, Bobo J, Habib A, et al. Characterization of neural mechanotransduction response in human traumatic brain injury organoid model. Sci Rep. 2023;13(1):13536. doi: 10.1038/s41598-023-40431-y
- Qian X, Nguyen HN, Song MM, et al. Brain-region-specific organoids using mini-bioreactors for modeling ZIKV exposure. Cell. 2016;165(5):1238-1254. doi: 10.1016/j.cell.2016.04.032
- Wang Z, Wang SN, Xu TY, et al. Cerebral organoids transplantation improves neurological motor function in rat brain injury. CNS Neurosci Ther. 2020;26(7):682-697. doi: 10.1111/cns.13286
- Gao X, Wang X, Xiong W, Chen J. In vivo reprogramming reactive glia into iPSCs to produce new neurons in the cortex following traumatic brain injury. Sci Rep. 2016;6:22490. doi: 10.1038/srep22490
- Kim JT, Kim TY, Youn DH, et al. Human embryonic stem cell-derived cerebral organoids for treatment of mild traumatic brain injury in a mouse model. Biochem Biophys Res Commun. 2022;635:169-178. doi: 10.1016/j.bbrc.2022.10.045
- Lim SH, Jung H, Youn DH, et al. Mild traumatic brain injury and subsequent acute pulmonary inflammatory response. J Korean Neurosurg Soc. 2022;65(5):680-687. doi: 10.3340/jkns.2021.0310
- Kim JT, Song K, Han SW, et al. Modeling of the brain-lung axis using organoids in traumatic brain injury: An updated review. Cell Biosci. 2024;14(1):83. doi: 10.1186/s13578-024-01252-2
- Wang P, Jin L, Zhang M, et al. Blood-brain barrier injury and neuroinflammation induced by SARS-CoV-2 in a lung-brain microphysiological system. Nat Biomed Eng. 2024;8(8):1053-1068. doi: 10.1038/s41551-023-01054-w
- Popernack ML, Gray N, Reuter-Rice K. Moderate-to-severe traumatic brain injury in children: Complications and rehabilitation strategies. J Pediatr Health Care. 2015;29(3):e1-e7. doi: 10.1016/j.pedhc.2014.09.003
- Erices JI, Bizama C, Niechi I, et al. Glioblastoma microenvironment and invasiveness: New insights and therapeutic targets. Int J Mol Sci. 2023;24(8):7047. doi: 10.3390/ijms24087047
- Bikfalvi A, da Costa CA, Avril T, et al. Challenges in glioblastoma research: Focus on the tumor microenvironment. Trends Cancer. 2023;9(1):9-27. doi: 10.1016/j.trecan.2022.09.005
- Tchoghandjian A, Baeza-Kallee N, Beclin C, et al. Cortical and subventricular zone glioblastoma-derived stem-like cells display different molecular profiles and differential in vitro and in vivo properties. Ann Surg Oncol. 2012;19(Suppl 3):S608-S619. doi: 10.1245/s10434-011-2093-5
- Carlson JC, Cantu Gutierrez M, Lozzi B, et al. Identification of diverse tumor endothelial cell populations in malignant glioma. Neuro Oncol. 2021;23(6):932-944. doi: 10.1093/neuonc/noaa297
- Jhaveri N, Chen TC, Hofman FM. Tumor vasculature and glioma stem cells: Contributions to glioma progression. Cancer Lett. 2016;380(2):545-551. doi: 10.1016/j.canlet.2014.12.028
- Cui J, Wang X, Li J, et al. Immune exosomes loading self-assembled nanomicelles traverse the blood-brain barrier for chemo-immunotherapy against glioblastoma. ACS Nano. 2023. doi: 10.1021/acsnano.2c10219
- Avril T, Vauleon E, Tanguy-Royer S, Mosser J, Quillien V. Mechanisms of immunomodulation in human glioblastoma. Immunotherapy. 2011;3(4 Suppl):42-44. doi: 10.2217/imt.11.39
- Friese MA, Platten M, Lutz SZ, et al. MICA/NKG2D-mediated immunogene therapy of experimental gliomas. Cancer Res. 2003;63(24):8996-9006.
- Kepp O, Loos F, Liu P, Kroemer G. Extracellular nucleosides and nucleotides as immunomodulators. Immunol Rev. 2017;280(1):83-92. doi: 10.1111/imr.12571
- Shahar T, Rozovski U, Hess KR, et al. Percentage of mesenchymal stem cells in high-grade glioma tumor samples correlates with patient survival. Neuro Oncol. 2017;19(5):660-668. doi: 10.1093/neuonc/now239
- Autier L, Clavreul A, Cacicedo ML, et al. A new glioblastoma cell trap for implantation after surgical resection. Acta Biomater. 2019;84:268-279. doi: 10.1016/j.actbio.2018.11.027
- Kasapidou PM, de Montullé EL, Dembélé KP, et al. Hyaluronic acid-based hydrogels loaded with chemoattractant and anticancer drug - new formulation for attracting and tackling glioma cells. Soft Matter. 2021;17(48):10846-10861. doi: 10.1039/d1sm01003d
- Gould SE, Junttila MR, de Sauvage FJ. Translational value of mouse models in oncology drug development. Nat Med. 2015;21(5):431-439. doi: 10.1038/nm.3853
- Perrin S. Preclinical research: Make mouse studies work. Nature. 2014;507(7493):423-425. doi: 10.1038/507423a
- Pollard SM, Yoshikawa K, Clarke ID, et al. Glioma stem cell lines expanded in adherent culture have tumor-specific phenotypes and are suitable for chemical and genetic screens. Cell Stem Cell. 2009;4(6):568-580. doi: 10.1016/j.stem.2009.03.014
- Pasupuleti V, Vora L, Prasad R, Nandakumar DN, Khatri DK. Glioblastoma preclinical models: Strengths and weaknesses. Biochim Biophys Acta Rev Cancer. 2024;1879(1):189059. doi: 10.1016/j.bbcan.2023.189059
- Vaubel RA, Tian S, Remonde D, et al. Genomic and phenotypic characterization of a broad panel of patient-derived xenografts reflects the diversity of glioblastoma. Clin Cancer Res. 2020;26(5):1094-1104. doi: 10.1158/1078-0432.CCR-19-0909
- Golebiewska A, Hau AC, Oudin A, et al. Patient-derived organoids and orthotopic xenografts of primary and recurrent gliomas represent relevant patient avatars for precision oncology. Acta Neuropathol. 2020;140(6):919-949. doi: 10.1007/s00401-020-02226-7
- Hubert CG, Rivera M, Spangler LC, et al. A three-dimensional organoid culture system derived from human glioblastomas recapitulates the hypoxic gradients and cancer stem cell heterogeneity of tumors found in vivo. Cancer Res. 2016;76(8):2465-2477. doi: 10.1158/0008-5472.CAN-15-2402
- Jacob F, Salinas RD, Zhang DY, et al. A patient-derived glioblastoma organoid model and biobank recapitulates inter- and intra-tumoral heterogeneity. Cell. 2020;180(1):188- 204.e22. doi: 10.1016/j.cell.2019.11.036
- Lu P, Weaver VM, Werb Z. The extracellular matrix: A dynamic niche in cancer progression. J Cell Biol. 2012;196(4):395-406. doi: 10.1083/jcb.201102147
- Quail DF, Joyce JA. The microenvironmental landscape of brain tumors. Cancer Cell. 2017;31(3):326-341. doi: 10.1016/j.ccell.2017.02.009
- Linkous A, Balamatsias D, Snuderl M, et al. Modeling patient-derived glioblastoma with cerebral organoids. Cell Rep. 2019;26(12):3203-3211.e5. doi: 10.1016/j.celrep.2019.02.063
- Weth FR, Peng L, Paterson E, Tan ST, Gray C. Utility of the cerebral organoid glioma ‘GLICO’ model for screening applications. Cells. 2022;12(1):153. doi: 10.3390/cells12010153
- Louis DN, Perry A, Reifenberger G, et al. The 2016 World Health Organization classification of tumors of the central nervous system: A summary. Acta Neuropathol. 2016;131(6):803-820. doi: 10.1007/s00401-016-1545-1
- Zhang C, Jin M, Zhao J, Chen J, Jin W. Organoid models of glioblastoma: Advances, applications and challenges. Am J Cancer Res. 2020;10(8):2242-2257.
- Bian S, Repic M, Guo Z, et al. Genetically engineered cerebral organoids model brain tumor formation. Nat Methods. 2018;15(8):631-639. doi: 10.1038/s41592-018-0070-7
- Ogawa J, Pao GM, Shokhirev MN, Verma IM. Glioblastoma model using human cerebral organoids. Cell Rep. 2018;23(4):1220-1229. doi: 10.1016/j.celrep.2018.03.105
- Zhang L, Liu F, Weygant N, et al. A novel integrated system using patient-derived glioma cerebral organoids and xenografts for disease modeling and drug screening. Cancer Lett. 2021;500:87-97. doi: 10.1016/j.canlet.2020.12.013
- Soto C, Pritzkow S. Protein misfolding, aggregation, and conformational strains in neurodegenerative diseases. Nat Neurosci. 2018;21(10):1332-1340. doi: 10.1038/s41593-018-0235-9
- Wray S. Modelling neurodegenerative disease using brain organoids. Semin Cell Dev Biol. 2021;111:60-66. doi: 10.1016/j.semcdb.2020.05.012
- Van Vliet EF, Knol MJ, Schiffelers RM, Caiazzo M, Fens MHAM. Levodopa-loaded nanoparticles for the treatment of Parkinson’s disease. J Control Release. 2023;360:212-224. doi: 10.1016/j.jconrel.2023.06.026
- Tagliaferro P, Burke RE. Retrograde axonal degeneration in Parkinson disease. J Parkinsons Dis. 2016;6(1):1-15. doi: 10.3233/JPD-150769
- Ye H, Robak LA, Yu M, Cykowski M, Shulman JM. Genetics and pathogenesis of Parkinson’s syndrome. Annu Rev Pathol. 2023;18:95-121. doi: 10.1146/annurev-pathmechdis-031521-034145
- Burbulla LF, Song P, Mazzulli JR, et al. Dopamine oxidation mediates mitochondrial and lysosomal dysfunction in Parkinson’s disease. Science. 2017;357(6357):1255-1261. doi: 10.1126/science.aam9080
- Bartels T, De Schepper S, Hong S. Microglia modulate neurodegeneration in Alzheimer’s and Parkinson’s diseases. Science. 2020;370(6512):66-69. doi: 10.1126/science.abb8587
- Lan G, Wang P, Chan RB, et al. Astrocytic VEGFA: An essential mediator in blood-brain-barrier disruption in Parkinson’s disease. Glia. 2022;70(2):337-353. doi: 10.1002/glia.24109
- Kam TI, Hinkle JT, Dawson TM, Dawson VL. Microglia and astrocyte dysfunction in Parkinson’s disease. Neurobiol Dis. 2020;144:105028. doi: 10.1016/j.nbd.2020.105028
- Isik S, Yeman Kiyak B, Akbayir R, Seyhali R, Arpaci T. Microglia Mediated neuroinflammation in Parkinson’s disease. Cells. 2023;12(7):1012. doi: 10.3390/cells12071012
- Morris HR, Spillantini MG, Sue CM, Williams-Gray CH. The pathogenesis of Parkinson’s disease. Lancet. 2024;403(10423):293-304. doi: 10.1016/S0140-6736(23)01478-2
- Nalls MA, Blauwendraat C, Vallerga CL, et al. Identification of novel risk loci, causal insights, and heritable risk for Parkinson’s disease: A meta-analysis of genome-wide association studies. Lancet Neurol. 2019;18(12):1091-1102. doi: 10.1016/S1474-4422(19)30320-5
- Pan H, Liu Z, Ma J, et al. Genome-wide association study using whole-genome sequencing identifies risk loci for Parkinson’s disease in Chinese population. NPJ Parkinsons Dis. 2023;9(1):22. doi: 10.1038/s41531-023-00456-6
- Bloem BR, Okun MS, Klein C. Parkinson’s disease. Lancet. 2021;397(10291):2284-2303. doi: 10.1016/S0140-6736(21)00218-X
- Takahashi K, Yamanaka S. Induction of pluripotent stem cells from mouse embryonic and adult fibroblast cultures by defined factors. Cell. 2006;126(4):663-676. doi: 10.1016/j.cell.2006.07.024
- Yu J, Vodyanik MA, Smuga-Otto K, et al. Induced pluripotent stem cell lines derived from human somatic cells. Science. 2007;318(5858):1917-1920. doi: 10.1126/science.1151526
- Garcia-Leon JA, Vitorica J, Gutierrez A. Use of human pluripotent stem cell-derived cells for neurodegenerative disease modeling and drug screening platform. Future Med Chem. 2019;11(11):1305-1322. doi: 10.4155/fmc-2018-0520
- Mazzulli JR, Zunke F, Isacson O, Studer L, Krainc D. α-Synuclein-induced lysosomal dysfunction occurs through disruptions in protein trafficking in human midbrain synucleinopathy models. Proc Natl Acad Sci U S A. 2016;113(7):1931-1936. doi: 10.1073/pnas.1520335113
- Mazzulli JR, Zunke F, Tsunemi T, et al. Activation of β-glucocerebrosidase reduces pathological α-synuclein and restores lysosomal function in Parkinson’s patient midbrain neurons. J Neurosci. 2016;36(29):7693-7706. doi: 10.1523/JNEUROSCI.0628-16.2016
- Kim S, Yun SP, Lee S, et al. GBA1 deficiency negatively affects physiological α-synuclein tetramers and related multimers. Proc Natl Acad Sci U S A. 2018;115(4):798-803. doi: 10.1073/pnas.1700465115
- Lei T, Zhang X, Fu G, et al. Advances in human cellular mechanistic understanding and drug discovery of brain organoids for neurodegenerative diseases. Ageing Res Rev. 2024;102:102517. doi: 10.1016/j.arr.2024.102517
- Bolognin S, Fossépré M, Qing X, et al. 3D cultures of Parkinson’s disease-specific dopaminergic neurons for high content phenotyping and drug testing. Adv Sci (Weinh). 2018;6(1):1800927. doi: 10.1002/advs.201800927
- Pereira JD, DuBreuil DM, Devlin AC, et al. Human sensorimotor organoids derived from healthy and amyotrophic lateral sclerosis stem cells form neuromuscular junctions. Nat Commun. 2021;12(1):4744. doi: 10.1038/s41467-021-24776-4
- Burbulla LF, Jeon S, Zheng J, Song P, Silverman RB, Krainc D. A modulator of wild-type glucocerebrosidase improves pathogenic phenotypes in dopaminergic neuronal models of Parkinson’s disease. Sci Transl Med. 2019;11(514):eaau6870. doi: 10.1126/scitranslmed.aau6870
- Zheng J, Jeon S, Jiang W, et al. Conversion of quinazoline modulators from inhibitors to activators of β-glucocerebrosidase. J Med Chem. 2019;62(3):1218-1230. doi: 10.1021/acs.jmedchem.8b01294
- Smits LM, Reinhardt L, Reinhardt P, et al. Modeling Parkinson’s disease in midbrain-like organoids. NPJ Parkinsons Dis. 2019;5:5. doi: 10.1038/s41531-019-0078-4
- Babu HWS, Kumar SM, Kaur H, Iyer M, Vellingiri B. Midbrain organoids for Parkinson’s disease (PD) - A powerful tool to understand the disease pathogenesis. Life Sci. 2024;345:122610. doi: 10.1016/j.lfs.2024.122610
- Arenas E. Wnt signaling in midbrain dopaminergic neuron development and regenerative medicine for Parkinson’s disease. J Mol Cell Biol. 2014;6(1):42-53. doi: 10.1093/jmcb/mju001
- Kirkeby A, Grealish S, Wolf DA, et al. Generation of regionally specified neural progenitors and functional neurons from human embryonic stem cells under defined conditions. Cell Rep. 2012;1(6):703-714. doi: 10.1016/j.celrep.2012.04.009
- Shang J, Li B, Fan H, et al. Sevoflurane promotes premature differentiation of dopaminergic neurons in hiPSC-derived midbrain organoids. Front Cell Dev Biol. 2022;10:941984. doi: 10.3389/fcell.2022.941984
- Ma C, Seong H, Li X, Yu X, Xu S, Li Y. Human brain organoid: A versatile tool for modeling neurodegeneration diseases and for drug screening. Stem Cells Int. 2022;2022:2150680. doi: 10.1155/2022/2150680
- Singleton AB, Farrer M, Johnson J, et al. alpha-Synuclein locus triplication causes Parkinson’s disease. Science. 2003;302(5646):841. doi: 10.1126/science.1090278
- Kim H, Park HJ, Choi H, et al. Modeling G2019S-LRRK2 sporadic Parkinson’s disease in 3D midbrain organoids. Stem Cell Reports. 2019;12(3):518-531. doi: 10.1016/j.stemcr.2019.01.020
- Wulansari N, Darsono WHW, Woo HJ, et al. Neurodevelopmental defects and neurodegenerative phenotypes in human brain organoids carrying Parkinson’s disease-linked DNAJC6 mutations. Sci Adv. 2021;7(8):eabb1540. doi: 10.1126/sciadv.abb1540. Erratum in: Sci Adv. 2023;9(44):eadl1498. doi: 10.1126/sciadv.adl1498
- Jarazo J, Barmpa K, Modamio J, et al. Parkinson’s disease phenotypes in patient neuronal cultures and brain organoids improved by 2-hydroxypropyl-β-cyclodextrin treatment. Mov Disord. 2022;37(1):80-94. doi: 10.1002/mds.28810
- Zagare A, Barmpa K, Smajic S, et al. Midbrain organoids mimic early embryonic neurodevelopment and recapitulate LRRK2-p.Gly2019Ser-associated gene expression. Am J Hum Genet. 2022;109(2):311-327. doi: 10.1016/j.ajhg.2021.12.009
- Mohamed NV, Sirois J, Ramamurthy J, et al. Midbrain organoids with an SNCA gene triplication model key features of synucleinopathy. Brain Commun. 2021;3(4):fcab223. doi: 10.1093/braincomms/fcab223
- Jo J, Yang L, Tran HD, et al. Lewy body-like inclusions in human midbrain organoids carrying glucocerebrosidase and α-synuclein mutations. Ann Neurol. 2021;90(3):490-505. doi: 10.1002/ana.26166
- Becerra-Calixto A, Mukherjee A, Ramirez S, et al. Lewy body-like pathology and loss of dopaminergic neurons in midbrain organoids derived from familial Parkinson’s disease patient. Cells. 2023;12(4):625. doi: 10.3390/cells12040625
- Zheng X, Han D, Liu W, et al. Human iPSC-derived midbrain organoids functionally integrate into striatum circuits and restore motor function in a mouse model of Parkinson’s disease. Theranostics. 2023;13(8):2673-2692. doi: 10.7150/thno.80271