Nerve–bone crosstalk manipulates bone organoid development and bone regeneration: A review and perspectives
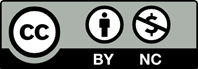
As an innovative regenerative medicine technology, bone organoids represent a promising therapy for refractory bone injury repair, whereas the key to fabricating bone organoids is grounded in the utilization of biomaterials with osteogenesis cues. Considering the intricate crosstalk between neurons and osteocytes would support bone organoid development and bone wound healing, it is extremely essential to predicate biomaterial design and osteo-organoid construction on understanding the roles of neural growth in ossification center formation and bone-like tissue development. Therefore, this review presents the recent advances of bone organoids with innervated ossification centers after the detailed introduction in the nerve–bone crosstalk. Beginning with the exploration of underlying interaction mechanisms within the osteogenic microenvironment, the importance of the nerve–bone crosstalk on skeleton development and bone regeneration is emphasized at first. The following discussions mainly include diverse biomaterial strategies designed to enhance osteogenesis through early innervation, such as the incorporation of bioactive minerals, controlled release of neurotrophic factors, and exosome-mediated nerve regeneration. Last but not least, the review highlights the advanced technologies in fabricating tissue-engineered bone organoids, with a focus on the applications of cell-laden and multicellular 3D-bioprinted bone microtissues for constructing bone organoids with neurovascularization. These insights are critical to the development of novel biomaterials to construct innervated osteo-organoids, with significant implications for future clinical applications, while also exploring the potential to advance bone regenerative therapies through enhanced nerve–bone crosstalk and laying the foundation for innovative translational research in this field.

- Wang W, Yeung KWK. Bone grafts and biomaterials substitutes for bone defect repair: A review. Bioact Mater. 2017;2(4):224-247. doi: 10.1016/j.bioactmat.2017.05.007
- Miron RJ, Gruber R, Hedbom E, et al. Impact of bone harvesting techniques on cell viability and the release of growth factors of autografts. Clin Implant Dent Relat Res. 2013;15(4):481-489. doi: 10.1111/j.1708-8208.2012.00440.x
- Giannoudis PV, Dinopoulos H, Tsiridis E. Bone substitutes: An update. Injury. 2005;36 Suppl 3:S20-S27. doi: 10.1016/j.injury.2005.07.029
- Vidal L, Kampleitner C, Brennan MA, Hoornaert A, Layrolle P. Reconstruction of large skeletal defects: Current clinical therapeutic strategies and future directions using 3D printing. Front Bioeng Biotechnol. 2020;8:61. doi: 10.3389/fbioe.2020.00061
- Hall GN, Tam WL, Andrikopoulos KS, et al. Patterned, organoid-based cartilaginous implants exhibit zone specific functionality forming osteochondral-like tissues in vivo. Biomaterials. 2021;273:120820. doi: 10.1016/j.biomaterials.2021.120820
- Xie C, Liang R, Ye J, et al. High-efficient engineering of osteo-callus organoids for rapid bone regeneration within one month. Biomaterials. 2022;288:121741. doi: 10.1016/j.biomaterials.2022.121741
- Luo M, Zhao Z, Yi J. Osteogenesis of bone marrow mesenchymal stem cell in hyperglycemia. Front Endocrinol (Lausanne). 2023;14:1150068. doi: 10.3389/fendo.2023.1150068
- Dec P, Modrzejewski A, Pawlik A. Existing and novel biomaterials for bone tissue engineering. Int J Mol Sci. 2022;24(1):529. doi: 10.3390/ijms24010529
- Arthur A, Gronthos S. Clinical application of bone marrow mesenchymal stem/stromal cells to repair skeletal tissue. Int J Mol Sci. 2020;21(24):9759. doi: 10.3390/ijms21249759
- Rajpar I, Tomlinson RE. Function of peripheral nerves in the development and healing of tendon and bone. Semin Cell Dev Biol. 2022;123:48-56. doi: 10.1016/j.semcdb.2021.05.001
- Gugjoo MB, Amarpal, Abdelbaset-Ismail A, et al. Mesenchymal stem cells with IGF-1 and TGF- beta1 in laminin gel for osteochondral defects in rabbits. Biomed Pharmacother. 2017;93:1165-1174. doi: 10.1016/j.biopha.2017.07.032
- Liu X, Gaihre B, George MN, et al. 3D bioprinting of oligo (poly[ethylene glycol] fumarate) for bone and nerve tissue engineering. J Biomed Mater Res A. 2021;109(1):6-17. doi: 10.1002/jbm.a.37002
- Elefteriou F. Impact of the autonomic nervous system on the skeleton. Physiol Rev. 2018;98(3):1083-1112. doi: 10.1152/physrev.00014.2017
- Fu S, Mei G, Wang Z, et al. Neuropeptide substance P improves osteoblastic and angiogenic differentiation capacity of bone marrow stem cells in vitro. Biomed Res Int. 2014;2014:596023. doi: 10.1155/2014/596023
- Yahara M, Tei K, Tamura M. Inhibition of neuropeptide Y Y1 receptor induces osteoblast differentiation in MC3T3-E1 cells. Mol Med Rep. 2017;16(3):2779-2784. doi: 10.3892/mmr.2017.6866
- Xu Y, Xia M, Chen T, et al. Inferior alveolar nerve transection disturbs innate immune responses and bone healing after tooth extraction. Ann N Y Acad Sci. 2019;1448(1):52-64. doi: 10.1111/nyas.14120
- Adameyko I, Ernfors P. Nerves do it again: Donation of mesenchymal cells for tissue regeneration. Cell Stem Cell. 2019;24(2):195-197. doi: 10.1016/j.stem.2019.01.006
- Sayilekshmy M, Hansen RB, Delaisse JM, Rolighed L, Andersen TL, Heegaard AM. Innervation is higher above bone remodeling surfaces and in cortical pores in human bone: Lessons from patients with primary hyperparathyroidism. Sci Rep. 2019;9(1):5361. doi: 10.1038/s41598-019-41779-w
- Cooper RR. Nerves in cortical bone. Science. 1968;160(3825):327-328. doi: 10.1126/science.160.3825.327
- Hohmann EL, Elde RP, Rysavy JA, Einzig S, Gebhard RL. Innervation of periosteum and bone by sympathetic vasoactive intestinal peptide-containing nerve fibers. Science. 1986;232(4752):868-871. doi: 10.1126/science.3518059
- Jonnakuty C, Gragnoli C. What do we know about serotonin? J Cell Physiol. 2008;217(2):301-306. doi: 10.1002/jcp.21533
- van der Klaauw AA, Croizier S, Mendes de Oliveira E, et al. Human semaphorin 3 variants link melanocortin circuit development and energy balance. Cell. 2019;176(4):729-742.e718. doi: 10.1016/j.cell.2018.12.009
- Sato S, Hanada R, Kimura A, et al. Central control of bone remodeling by neuromedin U. Nat Med. 2007;13(10):1234-1240. doi: 10.1038/nm1640
- Bellier JP, Kimura H. Peripheral type of choline acetyltransferase: Biological and evolutionary implications for novel mechanisms in cholinergic system. J Chem Neuroanat. 2011;42(4):225-235. doi: 10.1016/j.jchemneu.2011.02.005
- Hill EL, Elde R. Distribution of CGRP-, VIP-, D beta H-, SP-, and NPY-immunoreactive nerves in the periosteum of the rat. Cell Tissue Res. 1991;264(3):469-480. doi: 10.1007/BF00319037
- Uddin M, Shibata H. Distribution of neuronal structures immunoreactive for parvalbumin in the midcingulate cortex of the rabbit. Anat Histol Embryol. 2020;49(1):150-156. doi: 10.1111/ahe.12503
- Tanaka A, Kaku N, Tabata T, Tagomori H, Tsumura H. Comparison of early femoral bone remodeling and functional outcome after total hip arthroplasty using the SL-PLUS MIA stem with and without hydroxyapatite coating. Musculoskelet Surg. 2020;104(3):313-320. doi: 10.1007/s12306-019-00622-1
- Li Z, Meyers CA, Chang L, et al. Fracture repair requires TrkA signaling by skeletal sensory nerves. J Clin Invest. 2019;129(12):5137-5150. doi: 10.1172/JCI128428
- Ye L, Xu J, Mi J, et al. Biodegradable magnesium combined with distraction osteogenesis synergistically stimulates bone tissue regeneration via CGRP-FAK-VEGF signaling axis. Biomaterials. 2021;275:120984. doi: 10.1016/j.biomaterials.2021.120984
- Tao R, Mi B, Hu Y, et al. Hallmarks of peripheral nerve function in bone regeneration. Bone Res. 2023;11(1):6. doi: 10.1038/s41413-022-00240-x
- Gordon T. Peripheral nerve regeneration and muscle reinnervation. Int J Mol Sci. 2020;21(22):8652. doi: 10.3390/ijms21228652
- Yang S, Wang C, Zhu J, et al. Self-assembling peptide hydrogels functionalized with LN- and BDNF- mimicking epitopes synergistically enhance peripheral nerve regeneration. Theranostics. 2020;10(18):8227-8249. doi: 10.7150/thno.44276
- Sun S, Diggins NH, Gunderson ZJ, Fehrenbacher JC, White FA, Kacena MA. No pain, no gain? The effects of pain-promoting neuropeptides and neurotrophins on fracture healing. Bone. 2020;131:115109. doi: 10.1016/j.bone.2019.115109
- Xu J, Zhang Z, Zhao J, et al. Interaction between the nervous and skeletal systems. Front Cell Dev Biol. 2022;10:976736. doi: 10.3389/fcell.2022.976736
- Gu X, Huang C, Wang S, et al. Transcriptomic analysis of the rat dorsal root ganglion after fracture. Mol Neurobiol. 2024;61(3):1467-1478. doi: 10.1007/s12035-023-03637-9
- Xu H, Tian F, Liu Y, et al. Magnesium malate-modified calcium phosphate bone cement promotes the repair of vertebral bone defects in minipigs via regulating CGRP. J Nanobiotechnology. 2024;22(1):368. doi: 10.1186/s12951-024-02595-1
- Wan QQ, Qin WP, Ma YX, et al. Crosstalk between bone and nerves within bone. Adv Sci (Weinh). 2021;8(7):2003390. doi: 10.1002/advs.202003390
- Takeda S, Elefteriou F, Levasseur R, et al. Leptin regulates bone formation via the sympathetic nervous system. Cell. 2002;111(3):305-317. doi: 10.1016/s0092-8674(02)01049-8
- Elefteriou F, Ahn JD, Takeda S, et al. Leptin regulation of bone resorption by the sympathetic nervous system and CART. Nature. 2005;434(7032):514-520. doi: 10.1038/nature03398
- Yadav VK, Oury F, Suda N, et al. A serotonin-dependent mechanism explains the leptin regulation of bone mass, appetite, and energy expenditure. Cell. 2009;138(5):976-989. doi: 10.1016/j.cell.2009.06.051
- Levi-Montalcini R. The nerve growth factor 35 years later. Science. 1987;237(4819):1154-1162. doi: 10.1126/science.3306916
- Tomlinson RE, Li Z, Li Z, et al. NGF-TrkA signaling in sensory nerves is required for skeletal adaptation to mechanical loads in mice. Proc Natl Acad Sci U S A. 2017;114(18):E3632-E3641. doi: 10.1073/pnas.1701054114
- Yada M, Yamaguchi K, Tsuji T. NGF stimulates differentiation of osteoblastic MC3T3-E1 cells. Biochem Biophys Res Commun. 1994;205(2):1187-1193. doi: 10.1006/bbrc.1994.2791
- Zheng MG, Sui WY, He ZD, et al. TrkA regulates the regenerative capacity of bone marrow stromal stem cells in nerve grafts. Neural Regen Res. 2019;14(10):1765-1771. doi: 10.4103/1673-5374.257540
- Wang F, Yuan K, Zhou X, et al. Nerve growth factors promotes osteogenic differentiation through TGF-β and BMP-9 signaling pathways in bone mesenchymal stem cells. J Biomater Tissue Eng. 2020;10(1):46-52. doi: 10.1166/jbt.2020.2199
- Wang L, Cao J, Lei DL, et al. Application of nerve growth factor by gel increases formation of bone in mandibular distraction osteogenesis in rabbits. Br J Oral Maxillofac Surg. 2010;48(7):515-519. doi: 10.1016/j.bjoms.2009.08.042
- Eppley BL, Snyders RV, Winkelmann TM, Roufa DG. Efficacy of nerve growth factor in regeneration of the mandibular nerve: A preliminary report. J Oral Maxillofac Surg. 1991;49(1):61-68. doi: 10.1016/0278-2391(91)90268-q
- Park H, Poo MM. Neurotrophin regulation of neural circuit development and function. Nat Rev Neurosci. 2013;14(1):7-23. doi: 10.1038/nrn3379
- Ida-Yonemochi H, Yamada Y, Yoshikawa H, Seo K. Locally produced BDNF promotes sclerotic change in alveolar bone after nerve injury. PLoS One. 2017;12(1):e0169201. doi: 10.1371/journal.pone.0169201
- Ai LS, Sun CY, Zhang L, et al. Inhibition of BDNF in multiple myeloma blocks osteoclastogenesis via down-regulated stroma-derived RANKL expression both in vitro and in vivo. PLoS One. 2012;7(10):e46287. doi: 10.1371/journal.pone.0046287
- Su YW, Chung R, Ruan CS, et al. Neurotrophin-3 induces BMP-2 and VEGF activities and promotes the bony repair of injured growth plate cartilage and bone in rats. J Bone Miner Res. 2016;31(6):1258-1274. doi: 10.1002/jbmr.2786
- Kim BJ, Koh JM. Coupling factors involved in preserving bone balance. Cell Mol Life Sci. 2019;76(7):1243-1253. doi: 10.1007/s00018-018-2981-y
- Jeoung JY, Nam HY, Kwak J, et al. A decline in Wnt3a signaling is necessary for mesenchymal stem cells to proceed to replicative senescence. Stem Cells Dev. 2015;24(8):973-982. doi: 10.1089/scd.2014.0273
- Fukuda T, Takeda S, Xu R, et al. Sema3A regulates bone-mass accrual through sensory innervations. Nature. 2013;497(7450):490-493. doi: 10.1038/nature12115
- Janssen BJ, Robinson RA, Perez-Branguli F, et al. Structural basis of semaphorin-plexin signalling. Nature. 2010;467(7319):1118-1122. doi: 10.1038/nature09468
- Grässel SG. The role of peripheral nerve fibers and their neurotransmitters in cartilage and bone physiology and pathophysiology. Arthritis Res Ther. 2014;16(6):485. doi: 10.1186/s13075-014-0485-1
- Han J, Zou Z, Zhu C, et al. DNA synthesis of rat bone marrow mesenchymal stem cells through alpha1-adrenergic receptors. Arch Biochem Biophys. 2009;490(2):96-102. doi: 10.1016/j.abb.2009.08.009
- Mahns DA, Ivanusic JJ, Sahai V, Rowe MJ. An intact peripheral nerve preparation for monitoring the activity of single, periosteal afferent nerve fibres. J Neurosci Methods. 2006;156(1-2):140-144. doi: 10.1016/j.jneumeth.2006.02.019
- Hedderich J, El Bagdadi K, Angele P, et al. Norepinephrine inhibits the proliferation of human bone marrow-derived mesenchymal stem cells via beta2-adrenoceptor-mediated ERK1/2 and PKA phosphorylation. Int J Mol Sci. 2020;21(11):3824. doi: 10.3390/ijms21113924
- Shi L, Wang C, Yan Y, et al. Function study of vasoactive intestinal peptide on chick embryonic bone development. Neuropeptides. 2020;83:102077. doi: 10.1016/j.npep.2020.102077
- Hoover DB. Cholinergic modulation of the immune system presents new approaches for treating inflammation. Pharmacol Ther. 2017;179:1-16. doi: 10.1016/j.pharmthera.2017.05.002
- Bajayo A, Bar A, Denes A, et al. Skeletal parasympathetic innervation communicates central IL-1 signals regulating bone mass accrual. Proc Natl Acad Sci U S A. 2012;109(38):15455-15460. doi: 10.1073/pnas.1206061109
- Liu PS, Chen YY, Feng CK, Lin YH, Yu TC. Muscarinic acetylcholine receptors present in human osteoblast and bone tissue. Eur J Pharmacol. 2011;650(1):34-40. doi: 10.1016/j.ejphar.2010.09.031
- Papadopoulos DV, Kostas-Agnantis I, Gkiatas I, Tsantes AG, Ziara P, Korompilias AV. The role of new oral anticoagulants in orthopaedics: An update of recent evidence. Eur J Orthop Surg Traumatol. 2017;27(5):573-582. doi: 10.1007/s00590-017-1940-x
- Igwe JC, Jiang X, Paic F, et al. Neuropeptide Y is expressed by osteocytes and can inhibit osteoblastic activity. J Cell Biochem. 2009;108(3):621-630. doi: 10.1002/jcb.22294
- Corr A, Smith J, Baldock P. Neuronal control of bone remodeling. Toxicol Pathol. 2017;45(7):894-903. doi: 10.1177/0192623317738708
- Elefteriou F, Campbell P, Ma Y. Control of bone remodeling by the peripheral sympathetic nervous system. Calcif Tissue Int. 2014;94(1):140-151. doi: 10.1007/s00223-013-9752-4
- Yu W, Chen FC, Xu WN, et al. Inhibition of Y1 receptor promotes osteogenesis in bone marrow stromal cells via cAMP/PKA/CREB pathway. Front Endocrinol (Lausanne). 2020;11:583105. doi: 10.3389/fendo.2020.583105
- Lee NJ, Doyle KL, Sainsbury A, et al. Critical role for Y1 receptors in mesenchymal progenitor cell differentiation and osteoblast activity. J Bone Miner Res. 2010;25(8):1736-1747. doi: 10.1002/jbmr.61
- Wang L, Zhao R, Shi X, et al. Substance P stimulates bone marrow stromal cell osteogenic activity, osteoclast differentiation, and resorption activity in vitro. Bone. 2009;45(2):309-320. doi: 10.1016/j.bone.2009.04.203
- Cao J, Zhang S, Gupta A, et al. Sensory nerves affect bone regeneration in rabbit mandibular distraction osteogenesis. Int J Med Sci. 2019;16(6):831-837. doi: 10.7150/ijms.31883
- Hong HS, Lee J, Lee E, et al. A new role of substance P as an injury-inducible messenger for mobilization of CD29(+) stromal-like cells. Nat Med. 2009;15(4):425-435. doi: 10.1038/nm.1909
- Mu C, Hu Y, Hou Y, et al. Substance P-embedded multilayer on titanium substrates promotes local osseointegration via MSC recruitment. J Mater Chem B. 2020;8(6):1212-1222. doi: 10.1039/C9TB01124B
- Irie K, Hara-Irie F, Ozawa H, Yajima T. Calcitonin gene-related peptide (CGRP)-containing nerve fibers in bone tissue and their involvement in bone remodeling. Microsc Res Tech. 2002;58(2):85-90. doi: 10.1002/jemt.10122
- Takahashi N, Matsuda Y, Sato K, et al. Neuronal TRPV1 activation regulates alveolar bone resorption by suppressing osteoclastogenesis via CGRP. Sci Rep. 2016;6:29294. doi: 10.1038/srep29294
- Wang L, Shi X, Zhao R, et al. Calcitonin-gene-related peptide stimulates stromal cell osteogenic differentiation and inhibits RANKL induced NF-kappaB activation, osteoclastogenesis and bone resorption. Bone. 2010;46(5):1369-1379. doi: 10.1016/j.bone.2009.11.029
- Yang Z, Wu B, Jia S, et al. The mechanically activated p38/MMP-2 signaling pathway promotes bone marrow mesenchymal stem cell migration in rats. Arch Oral Biol. 2017;76:55-60. doi: 10.1016/j.archoralbio.2017.01.017
- Zhou R, Yuan Z, Liu J, Liu J. Calcitonin gene-related peptide promotes the expression of osteoblastic genes and activates the WNT signal transduction pathway in bone marrow stromal stem cells. Mol Med Rep. 2016;13(6):4689-4696. doi: 10.3892/mmr.2016.5117
- Mrak E, Guidobono F, Moro G, Fraschini G, Rubinacci A, Villa I. Calcitonin gene-related peptide (CGRP) inhibits apoptosis in human osteoblasts by beta-catenin stabilization. J Cell Physiol. 2010;225(3):701-708. doi: 10.1002/jcp.22266
- Cai XX, Luo E, Yuan Q. Interaction between Schwann cells and osteoblasts in vitro. Int J Oral Sci. 2010;2(2):74-81. doi: 10.4248/IJOS10039
- Faroni A, Mobasseri SA, Kingham PJ, Reid AJ. Peripheral nerve regeneration: Experimental strategies and future perspectives. Adv Drug Deliv Rev. 2015;82-83:160-167. doi: 10.1016/j.addr.2014.11.010
- Wu Z, Pu P, Su Z, Zhang X, Nie L, Chang Y. Schwann Cell-derived exosomes promote bone regeneration and repair by enhancing the biological activity of porous Ti6Al4V scaffolds. Biochem Biophys Res Commun. 2020;531(4):559-565. doi: 10.1016/j.bbrc.2020.07.094
- Xie M, Kamenev D, Kaucka M, et al. Schwann cell precursors contribute to skeletal formation during embryonic development in mice and zebrafish. Proc Natl Acad Sci U S A. 2019;116(30):15068-15073. doi: 10.1073/pnas.1900038116
- Xia W, Xie J, Cai Z, et al. Damaged brain accelerates bone healing by releasing small extracellular vesicles that target osteoprogenitors. Nat Commun. 2021;12(1):6043. doi: 10.1038/s41467-021-26302-y
- Guilherme A, Henriques F, Bedard AH, Czech MP. Molecular pathways linking adipose innervation to insulin action in obesity and diabetes mellitus. Nat Rev Endocrinol. 2019;15(4):207-225. doi: 10.1038/s41574-019-0165-y
- Zhou BO, Yue R, Murphy MM, Peyer JG, Morrison SJ. Leptin-receptor-expressing mesenchymal stromal cells represent the main source of bone formed by adult bone marrow. Cell Stem Cell. 2014;15(2):154-168. doi: 10.1016/j.stem.2014.06.008
- Carmeliet P, Tessier-Lavigne M. Common mechanisms of nerve and blood vessel wiring. Nature. 2005;436(7048):193-200. doi: 10.1038/nature03875
- Liu Q, Lei L, Yu T, Jiang T, Kang Y. Effect of brain-derived neurotrophic factor on the neurogenesis and osteogenesis in bone engineering. Tissue Eng Part A. 2018;24(15-16):1283-1292. doi: 10.1089/ten.TEA.2017.0462
- Fonseca TL, Jorgetti V, Costa CC, et al. Double disruption of alpha2A- and alpha2C-adrenoceptors results in sympathetic hyperactivity and high-bone-mass phenotype. J Bone Miner Res. 2011;26(3):591-603. doi: 10.1002/jbmr.243
- Tanaka K, Hirai T, Kodama D, Kondo H, Hamamura K, Togari A. α1B -Adrenoceptor signalling regulates bone formation through the up-regulation of CCAAT/enhancer-binding protein δ expression in osteoblasts. Br J Pharmacol. 2016;173(6):1058-1069. doi: 10.1111/bph.13418
- Moore RE, Smith CK 2nd, Bailey CS, Voelkel EF, Tashjian AH Jr. Characterization of beta-adrenergic receptors on rat and human osteoblast-like cells and demonstration that beta-receptor agonists can stimulate bone resorption in organ culture. Bone Miner. 1993;23(3):301-315. doi: 10.1016/s0169-6009(08)80105-5
- Heffner MA, Anderson MJ, Yeh GC, Genetos DC, Christiansen BA. Altered bone development in a mouse model of peripheral sensory nerve inactivation. J Musculoskelet Neuronal Interact. 2014;14(1):1-9.
- Hui T, Zhang GC, Feng DD, Ji P. Role of neuropeptide substance P and the bone morphogenetic protein signaling pathway in osteogenic differentiation of ST2 cells. Hua Xi Kou Qiang Yi Xue Za Zhi. 2018;36(4):378-383. doi: 10.7518/hxkq.2018.04.006
- Goto T, Nakao K, Gunjigake KK, Kido MA, Kobayashi S, Tanaka T. Substance P stimulates late-stage rat osteoblastic bone formation through neurokinin-1 receptors. Neuropeptides. 2007;41(1):25-31. doi: 10.1016/j.npep.2006.11.002
- Mei G, Zou Z, Fu S, et al. Substance P activates the Wnt signal transduction pathway and enhances the differentiation of mouse preosteoblastic MC3T3-E1 cells. Int J Mol Sci. 2014;15(4):6224-6240. doi: 10.3390/ijms15046224
- Marrella A, Lee TY, Lee DH, et al. Engineering vascularized and innervated bone biomaterials for improved skeletal tissue regeneration. Mater Today (Kidlington). 2018;21(4):362-376. doi: 10.1016/j.mattod.2017.10.005
- Vezenkova A, Locs J. Sudoku of porous, injectable calcium phosphate cements - Path to osteoinductivity. Bioact Mater. 2022;17:109-124. doi: 10.1016/j.bioactmat.2022.01.001
- No YJ, Xin X, Ramaswamy Y, et al. Novel injectable strontium-hardystonite phosphate cement for cancellous bone filling applications. Mater Sci Eng C Mater Biol Appl. 2019;97:103-115. doi: 10.1016/j.msec.2018.11.069
- Bai L, Song P, Su J. Bioactive elements manipulate bone regeneration. Biomater Transl. 2023;4(4):248-269. doi: 10.12336/biomatertransl.2023.04.005
- Li Q, Liu W, Hou W, et al. Micropatterned photothermal double-layer periosteum with angiogenesis-neurogenesis coupling effect for bone regeneration. Mater Today Bio. 2023;18:100536. doi: 10.1016/j.mtbio.2022.100536
- Zhang K, Hu H, Sun Y, et al. The bio-functionalized membrane loaded with Ta/WH nanoparticles promote bone regeneration through neurovascular coupling. Colloids Surf B Biointerfaces. 2023;230:113506. doi: 10.1016/j.colsurfb.2023.113506
- Huang J, Ma Y, Pang K, et al. Anisotropic microspheres-cryogel composites loaded with magnesium l-threonate promote osteogenesis, Angiogenesis, and neurogenesis for repairing bone defects. Biomacromolecules. 2023;24(7):3171-3183. doi: 10.1021/acs.biomac.3c00243
- Zhou P, Liu T, Liu W, et al. An antibacterial bionic periosteum with angiogenesis-neurogenesis coupling effect for bone regeneration. ACS Appl Mater Interfaces. 2024;16(16):21084- 21097. doi: 10.1021/acsami.4c01206
- Gao X, Murphy MM, Peyer JG, et al. Leptin receptor(+) cells promote bone marrow innervation and regeneration by synthesizing nerve growth factor. Nat Cell Biol. 2023;25(12):1746-1757. doi: 10.1038/s41556-023-01284-9
- Xu J, Li Z, Tower RJ, et al. NGF-p75 signaling coordinates skeletal cell migration during bone repair. Sci Adv. 2022;8(11):eabl5716. doi: 10.1126/sciadv.abl5716
- Wang X, Zheng W, Bai Z, et al. Mimicking bone matrix through coaxial electrospinning of core-shell nanofibrous scaffold for improving neurogenesis bone regeneration. Biomater Adv. 2023;145:213246. doi: 10.1016/j.bioadv.2022.213246
- Cheng C, Tang S, Cui S, et al. Nerve growth factor promote osteogenic differentiation of dental pulp stem cells through MEK/ERK signalling pathways. J Cell Mol Med. 2024;28(4):e18143. doi: 10.1111/jcmm.18143
- Zhang Z, Wang F, Huang X, et al. Engineered sensory nerve guides self-adaptive bone healing via NGF-TrkA signaling pathway. Adv Sci (Weinh). 2023;10(10):e2206155. doi: 10.1002/advs.202206155
- Fitzpatrick V, Martin-Moldes Z, Deck A, et al. Functionalized 3D-printed silk-hydroxyapatite scaffolds for enhanced bone regeneration with innervation and vascularization. Biomaterials. 2021;276:120995. doi: 10.1016/j.biomaterials.2021.120995
- Li W, Miao W, Liu Y, et al. Bioprinted constructs that mimic the ossification center microenvironment for targeted innervation in bone regeneration. Adv Funct Mater. 2022;32:2109871. doi: 10.1002/adfm.202109871
- Kubiak CA, Grochmal J, Kung TA, Cederna PS, Midha R, Kemp SWP. Stem-cell-based therapies to enhance peripheral nerve regeneration. Muscle Nerve. 2020;61(4):449-459. doi: 10.1002/mus.26760
- Burks SS, Diaz A, Haggerty AE, Oliva N, Midha R, Levi AD. Schwann cell delivery via a novel 3D collagen matrix conduit improves outcomes in critical length nerve gap repairs. J Neurosurg. 2021;135(4):1241-1251. doi: 10.3171/2020.8.JNS202349
- Han GH, Peng J, Liu P, et al. Therapeutic strategies for peripheral nerve injury: Decellularized nerve conduits and Schwann cell transplantation. Neural Regen Res. 2019;14(8):1343-1351. doi: 10.4103/1673-5374.253511
- Zhang X, Xiong Q, Lin W, et al. Schwann cells contribute to alveolar bone regeneration by promoting cell proliferation. J Bone Miner Res. 2023;38(1):119-130. doi: 10.1002/jbmr.4735
- Xu Y, Xu C, Song H, et al. Biomimetic bone-periosteum scaffold for spatiotemporal regulated innervated bone regeneration and therapy of osteosarcoma. J Nanobiotechnol. 2024;22(1):250. doi: 10.1186/s12951-024-02430-7
- Su Y, Zeng L, Deng R, et al. Endogenous electric field-coupled PD@BP biomimetic periosteum promotes bone regeneration through sensory nerve via fanconi anemia signaling pathway. Adv Healthc Mater. 2023;12(12):e2203027. doi: 10.1002/adhm.202203027
- Jing X, Xu C, Su W, et al. Photosensitive and conductive hydrogel induced innerved bone regeneration for infected bone defect repair. Adv Healthc Mater. 2023;12(3):e2201349. doi: 10.1002/adhm.202201349
- Wang T, Li W, Zhang Y, et al. Bioprinted constructs that simulate nerve-bone crosstalk to improve microenvironment for bone repair. Bioact Mater. 2023;27:377-393. doi: 10.1016/j.bioactmat.2023.02.013
- Hao Z, Ren L, Zhang Z, et al. A multifunctional neuromodulation platform utilizing Schwann cell-derived exosomes orchestrates bone microenvironment via immunomodulation, angiogenesis and osteogenesis. Bioact Mater. 2023;23:206-222. doi: 10.1016/j.bioactmat.2022.10.018
- Lian M, Qiao Z, Qiao S, et al. Nerve growth factor-preconditioned mesenchymal stem cell-derived exosome-functionalized 3D-printed hierarchical porous scaffolds with neuro-promotive properties for enhancing innervated bone regeneration. ACS Nano. 2024;18(10):7504-7520. doi: 10.1021/acsnano.3c11890
- Zhao D, Saiding Q, Li Y, Tang Y, Cui W. Bone organoids: Recent advances and future challenges. Adv Healthc Mater. 2024;13(5):e2302088. doi: 10.1002/adhm.202302088
- Cardier JE, Diaz-Solano D, Wittig O, et al. Osteogenic organoid for bone regeneration: Healing of bone defect in congenital pseudoarthrosis of the tibia. Int J Artif Organs. 2024;47(2):107-114. doi: 10.1177/03913988231220844
- Liu F, Wu Q, Liu Q, et al. Dental pulp stem cells-derived cannabidiol-treated organoid-like microspheroids show robust osteogenic potential via upregulation of WNT6. Commun Biol. 2024;7:972. doi: 10.1038/s42003-024-06655-y
- Zhang Y, Li G, Wang J, Zhou F, Ren X, Su J. Small joint organoids 3D bioprinting: Construction strategy and application. Small. 2024;20(8):e2302506. doi: 10.1002/smll.202302506
- Wang J, Yang Q, Saiding Q, et al. Geometric angles and gene expression in cells for structural bone regeneration. Adv Sci (Weinh). 2023;10(32):e2304111. doi: 10.1002/advs.202304111
- Zhang H, Zhang M, Zhai D, et al. Polyhedron-like biomaterials for innervated and vascularized bone regeneration. Adv Mater. 2023;35(42):e2302716. doi: 10.1002/adma.202302716
- Li S, Li Z, Yang J, Ha Y, Zhou X, He C. Inhibition of sympathetic activation by delivering calcium channel blockers from a 3D printed scaffold to promote bone defect repair. Adv Healthc Mater. 2022;11(16):e2200785. doi: 10.1002/adhm.202200785
- Guo S, He C. Bioprinted scaffold remodels the neuromodulatory microenvironment for enhancing bone regeneration. Adv Funct Mater. 2023;33:2304172. doi: 10.1002/adfm.202304172
- Zhao R, Shen Y, Deng X, et al. From brain to bone: Harnessing extracellular vesicles released from TBI to enhance osteogenesis by 3D-Printed hydrogel scaffold. Compos Part B Eng. 2023. doi: 10.1016/j.compositesb.2023.110909
- Joung D, Truong V, Neitzke CC, et al. 3D printed stem-cell derived neural progenitors generate spinal cord scaffolds. Adv Funct Mater. 2018;28(39):1801850. doi: 10.1002/adfm.201801850
- Zhang H, Qin C, Shi Z, et al. Bioprinting of inorganic-biomaterial/neural-stem-cell constructs for multiple tissue regeneration and functional recovery. Natl Sci Rev. 2024;11(4):nwae035. doi: 10.1093/nsr/nwae035
- Zhang M, Qin C, Wang Y, et al. 3D printing of tree-like scaffolds for innervated bone regeneration. Addit Manuf. 2022;54:102721. doi: 10.1016/j.addma.2022.102721
- Zhang H, Qin C, Zhang M, et al. Calcium silicate nanowires-containing multicellular bioinks for 3D bioprinting of neural-bone constructs. Nano Today. 2022;46:101584. doi: 10.1016/j.nantod.2022.101584
- Wang X, Jiang K, Zheng W, et al. Accelerated bone defect repairment by carbon nitride photoelectric conversion material in core–shell nanofibrous depended on neurogenesis. Chem Eng J. 2024;479:147360. doi: 10.1016/j.cej.2023.147360