Thermodynamic profiling during irreversible electroporation in porcine liver and pancreas: a case study series
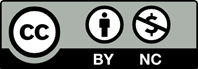
Aims: Firstly, to determine whether irreversible electroporation (IRE) is associated with heat generation in the liver and pancreas at clinical (≤ 1,500 V/cm) and supraclinical (> 1,500 V/cm) electroporation settings. Secondly, to assess the risk of thermal tissue damage in and adjacent to the treated volume in highly perfused versus moderately perfused parts of both organs. Thirdly, to investigate the influence of perfusion and of the presence and the orientation of a metal stent on the maximal thermal elevation (ΔTSession,max) in the tissue during an IRE session at fixed IRE settings. Finally, to determine whether the maximum temperature elevation within the IRE-subjected organ during an IRE treatment (single or multiple sessions) is reflected in the organ’s surface temperature.
Methods: The aims were investigated in 12 case studies conducted in 5 female Landrace pigs. Several IRE settings were applied for lateral (2), triangular (3), and rectangular (4) electrode configurations in the liver hilum, liver periphery, pancreas head, and pancreas tail. IRE series of 10 - 90 pulses were applied with pulse durations that varied from 70 µs - 90 µs and electric field strengths between 1,200 V/cm - 3,000 V/cm. In select cases, a metal stent was positioned in the bile duct at the level of the liver hilum. Temperatures were measured before, during, and after IRE in and adjacent to the treatment volumes using fiber optical temperature probes (temperature at the nucleation centers) and digital thermography (surface temperature). The occurrence of thermal damage was assumed to be at temperatures above 50 °C (ΔTSession,max ≥ 13 °C relative to body temperature of 37 °C). The temperature fluctuations at the organ surface (ΔTLocSurf) were compared to the maximum temperature elevation during an IRE treatment in the electroporation zone. In select cases, IRE was applied to tissue volumes encompassing the portal vein (PV) and a constricted and patent superior mesenteric vein (SMV) to determine the influence of the heatsink effect of PV and SMV on ΔTSession,max.
Results: The median baseline temperature was 31.6 °C - 36.3 °C. ΔTSession,max ranged from –1.7 °C - 25.5 °C in moderately perfused parts of the liver and pancreas, and from 0.0 °C - 5.8 °C in highly perfused parts. The median ΔTLocSurf of the liver and pancreas was 1.0 °C and 10.3 °C, respectively. Constricting the SMV in the pancreas head yielded a 0.8 °C higher ΔTSession,max. The presence of metal stents in the liver hilum resulted in a ΔTSession,max of 19.8 °C. Stents parallel to the electrodes caused a ΔTSession,max difference of 4.2 °C relative to the perpendicular orientation.
Conclusions: Depending on IRE settings and tissue type, IRE is capable of inducing considerable heating in the liver and pancreas that is sufficient to cause thermal tissue damage. More significant temperature elevations are positively correlated with increasing number of electrode pairs, electric field strength, and pulse number. Temperature elevations can be further exacerbated by the presence and orientation of metal stents. Temperature elevations at the nucleation centers are not always reflected in the organ’s surface temperature. Heat sink effects caused by large vessels were minimal in some instances, possibly due to reduced blood flow caused by anesthesia.
Relevance for patients: Appropriate IRE settings must be chosen based on the tissue type and the presence of stents to avoid thermal damage in healthy peritumoral tissue and to protect anatomical structures.
[1] Coelen RJ, Vogel JA, Vroomen LG, Roos E, Busch OR, van Delden OM, et al. Ablation with Irreversible Electroporation in Patients with Advanced Perihilar Cholangiocarcinoma (ALPACA): A Multicentre Phase I/II Feasibility Study Protocol. BMJ Open, 2017;7:e015810.
[2] Scheffer HJ, Vroomen LG, de Jong MC, Melenhorst MC, Zonderhuis BM, Daams F, et al. Ablation of Locally Advanced Pancreatic Cancer with Percutaneous Irreversible Electroporation: Results of the Phase I/II PANFIRE Study. Radiology 2017;282:585-97.
[3] Buijs M, Lienden KP, Wagstaff PG, Scheltema MJ, Bruin DM, Zondervan PJ, et al. Irreversible Electroporation for the Ablation of Renal Cell Carcinoma: A Prospective, Human, In Vivo Study Protocol (IDEAL Phase 2b). JMIR Res Protoc 2017;6:e21.
[4] Scheltema MJ, van den Bos W, de Bruin DM, Wijkstra H, Laguna MP, de Reijke TM. Focal vs Extended Ablation in Localized Prostate Cancer with Irreversible Electroporation; a Multi-center Randomized Controlled Trial. BMC Cancer 2016;16:299.
[5] O’Brien TJ, Bonakdar M, Bhonsle S, Neal RE 2nd, Aardema CH Jr., Robertson JL, et al. Effects of Internal Electrode Cooling on Irreversible Electroporation Using a Perfused Organ Model. Int J Hyperthermia 2019;35:44-55.
[6] Pucihar G, Kotnik T, Miklavcic D. Measuring the Induced Membrane Voltage with Di-8-ANEPPS. J Vis Exp 2009;33:1659.
[7] Neal RE, Davalos RV. The Feasibility of Irreversible Electroporation for the Treatment of Breast Cancer and Other Heterogeneous Systems. Ann Biomed Eng 2009;37:2615-25.
[8] Heger M, van der Wal AC, Storm G, van Gemert MJ. Potential Therapeutic Benefits Stemming from the Thermal Nature of Irreversible Electroporation of Solid Cancers. Hepatob Pancreatic Dis Int Lett 2015;14:331-3.
[9] Zhao J, Wen X, Tian L, Li T, Xu C, Wen X, et al. Irreversible Electroporation Reverses Resistance to Immune Checkpoint Blockade in Pancreatic Cancer. Nat Commun 2019;10:899.
[10] Lee EW, Thai S, Kee ST. Irreversible Electroporation: A Novel Image-Guided Cancer Therapy. Gut Liver Rev 2010;4:S99-104.
[11] Lee EW, Chen C, Prieto VE, Dry SM, Loh CT, Kee ST. Advanced Hepatic Ablation Technique for Creating Complete Cell Death: Irreversible Electroporation. Radiology 2010;255:426-33.
[12] OnikG, MikusP, Rubinsky B. Irreversible Electroporation: Implications for Prostate Ablation. Technol Cancer Res Treat 2007;6:295-300.
[13] van Gemert MJ, Wagstaff PG, de Bruin DM, van Leeuwen TG, van derWalAC, HegerM, et al. Irreversible Electroporation: Just Another Form of Thermal Therapy? Prostate 2015;75:332-5.
[14] Nickfarjam A, Firoozabadi SM. Parametric Study of Irreversible Electroporation with Different Needle Electrodes: Electrical and Thermal Analysis. Int J Hyperthermia 2014;30:335-47.
[15] Lopresto V, Pinto R, Farina L, Cavagnaro M. Microwave Thermal Ablation: Effects of Tissue Properties Variations on Predictive Models for Treatment Planning. Med Eng Phys 2017;46:63-70.
[16] Davalos RV, Mir LM, Rubinsky B. Tissue Ablation with Irreversible Electroporation. Ann Biomed Eng 2005;33:223-31.
[17] Dunki-Jacobs EM, Philips P, Martin RC. Evaluation of Thermal Injury to Liver, Pancreas and Kidney during Irreversible Electroporation in an In Vivo Experimental Model. Br J Surg 2014;101:1113-21.
[18] Cornelis FH, Cindrič H, Kos B, Fujimori M, Petre EN, Miklavčič D, et al. Peri-tumoral Metallic Implants Reduce the Efficacy of Irreversible Electroporation for the Ablation of Colorectal Liver Metastases. Cardiovasc Interv Radiol 2020;43:84-93.
[19] Faroja M, Ahmed M, Appelbaum L, Ben-David E, Moussa M, Sosna J, et al. Irreversible Electroporation Ablation: Is All the Damage Nonthermal? Radiology 2013;266:462-70.
[20] van den Bos W, Scheffer HJ, Vogel JA, Wagstaff PG, de Bruin DM, de Jong MC, et al. Thermal Energy during Irreversible Electroporation and the Influence of Different Ablation Parameters. J Vasc Interv Radiol 2016;27:433-43.
[21] Martin RC 2nd. Irreversible Electroporation of Stage 3 Locally Advanced Pancreatic Cancer: Optimal Technique and Outcomes. J Vis Surg 2015;1:4.
[22] O’Brien TJ, Lorenzo MF, Zhao Y, Neal Ii RE, Robertson JL, Goldberg SN, et al. Cycled Pulsing to Mitigate Thermal Damage for Multi-Electrode Irreversible Electroporation Therapy. Int J Hyperthermia 2019;36:953-63.
[23] Neal RE, Kavnoudias H, Thomson KR. An “off-the-shelf” System for Intraprocedural Electrical Current Evaluation and Monitoring of Irreversible Electroporation Therapy. Cardiovasc Intervent Radiol 2015;38:736-41.
[24] Ben-David E, Ahmed M, FarojaM, Moussa M, WandelA, Sosna J, et al. Irreversible Electroporation: Treatment Effect Is Susceptible to Local Environment and Tissue Properties. Radiology 2013;269:738-47.
[25] Wandel A, Ben-David E, Ulusoy BS, Neal R, Faruja M, Nissenbaum I, et al. Optimizing Irreversible Electroporation Ablation with a Bipolar Electrode. J Vasc Interv Radiol 2016;27:1441-50.
[26] Bardy GH, Coltorti F, Stewart RB, Greene HL, Ivey TD. Catheter-Mediated Electrical Ablation the Relation between Current and Pulse Width on Voltage Breakdown and Shock-Wave Generation. Circ Res 1988;63:409-14.
[27] Guenther E, Klein N, Mikus P, Stehling MK, Rubinsky B. Electrical Breakdown in Tissue Electroporation. Biochem Biophys Res Commun 2015;467:736-41.
[28] Edelblute CM, HornefJ, Burcus NI, Norman T, Beebe SJ, Schoenbach K, et al. Controllable Moderate Heating Enhances the Therapeutic Efficacy of Irreversible Electroporation for Pancreatic Cancer. Sci Rep 2017;7:11767.
[29] Rossmanna C, Haemmerich D. Review of Temperature Dependence of Thermal Properties, Dielectric Properties, and Perfusion of Biological Tissues at Hyperthermic and Ablation Temperatures. Crit Rev Biomed Eng Rev 2014;42:467-92.
[30] Scheffer HJ, Vogel JA, van den Bos W, Neal RE 2nd, van Lienden KP, Besselink MG, et al. The Influence of a Metal Stent on the Distribution of Thermal Energy during Irreversible Electroporation. PLoS One 2016;11:e0148457.
[31] Mansson C, Nilsson A, Karlson BM. Severe Complications with Irreversible Electroporation of the Pancreas in the Presence of a Metallic Stent: A Warning of a Procedure that Never Should be Performed. Acta Radiol Short Rep 2014;3:1–3.
[32] Haemmerich D, Schutt DJ, Wright AW, Webster JG, Mahvi DM. Electrical Conductivity Measurement of Excised Human Metastatic Liver Tumours before and after Thermal Ablation. Physiol Meas 2009;30:459-66.
[33] Stauffer PR, Rossetto F, Prakash M, Neuman DG, Lee T. Phantom and Animal Tissues for Modelling the Electrical Properties of Human Liver. Int J Hyperthermia 2003;19:89-101.
[34] Beitel-White N, Bhonsle S, Martin RC, Davalos RV. Electrical Characterization of Human Biological Tissue for Irreversible Electroporation Treatments. In: 2018 40th Annual International Conference of the IEEE Engineering in Medicine and Biology Society (EMBC); 2018. p. 4170-3.
[35] Marcan M, Kos B, Miklavcic D. Effect of Blood Vessel Segmentation on the Outcome of Electroporation-Based Treatments of Liver Tumors. PLoS One 2015;10:e0125591.
[36] Golberg A, BruinsmaBG,Uygun BE, Yarmush ML. Tissue Heterogeneity in Structure and Conductivity Contribute to Cell Survival during Irreversible Electroporation Ablation by “Electric Field Sinks” . Sci Rep 2014;5:8485.
[37] Zorbas G, Samaras T. A Study of the Sink Effect by Blood Vessels in Radiofrequency Ablation. Comput Biol Med 2015;57:182-6.
[38] Jiang K, Chen Y, Liu Y, Dong J, Liu A, Huang Z, et al. Heat- Irrigate Effect of Radiofrequency Ablation on Relevant Regional Hepatocyte in Living Swine Liver-Initial Study on Pathology. Cell Biochem Biophys 2015;72:37-41.
[39] Chen RD, Lu F, Wu F, Jiang T, Xie LT, Kong DX. An Analytical Solution for Temperature Distributions in Hepatic Radiofrequency Ablation Incorporating the Heat-sink Effect of Large Vessels. Phys Med Biol 2018;63:235026.
[40] Ringe KI, Lutat C, Rieder C, Schenk A, Wacker F, Raatschen HJ. Experimental Evaluation of the Heat Sink Effect in Hepatic Microwave Ablation. PLoS One, 2015;10:e0134301.