How the vagus nerve produces beat-to-beat heart rate variability; experiments in rabbits to mimic in vivo vagal patterns
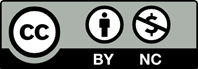
Background and Aim: Analysis of heart rate variability (HRV) has recently become the playing field of mathematicians and physicists, losing its relation to physiology and the clinic. To set the record straight, a set of animal experiments is presented here, which was designed to test how vagus nerve traffic might produce beat to beat (b-t-b) heart rate (HR) control, like the baroreflex will do in vivo.
Methods: The response of HR to vagus nerve stimulation was tested after bilateral vagotomy in rabbits under anesthesia. 3 Protocols were followed: 1. Single burst stimulation at varying moments in one cardiac cycle; 2. B-t-b stimulation in each cycle, coupled to the P-wave with variable delays; in addition, testing the effects of one increased or decreased burst; 3. Tetanic stimulation, shortly interrupted or increased at varying moments in the cardiac cycle.
Results and Conclusions: Sensitivity of the sinoatrial node to the timing of vagal bursts in its cycle from protocol 1 explains most of the observations. A single burst would be most effective when applied in late repolarization or early diastole of the sinoatrial node’s action potential. In b-t-b stimulation the longest cardiac cycles occur when bursts are timed just before the end of the ‘sensitive period’. Later coming bursts have their (diminished) effect on the next cycle; critically timed bursts induce an unstable HR, alternating between long and short cycles. This ran in synchrony with the respirator, thus producing a large respiratory sinus arrhythmia, even though the vagus nerves had been cut. HR-response to vagal burst activity shows two components: a fast one which is phase-sensitive and a slow one, that builds up with longer lasting activity and also disappears slowly. Tetanic stimulation results in prolonged, but variable cycle lengths which are difficult to change by short-lasting manipulation of impulse frequency, be it up or down.
Relevance for patients: Measurement of heart rate variability (HRV) and baroreflex sensitivity (BRS) have become clinical tools in the cardiology clinic and in hypertension research. This study shows how the underlying vagus nerve to heart rate physiology is responsible for moment-to-moment variability in these numbers at almost unchanged underlying physiology. Programmed stimulation of the vagus nerves in acute animals (rabbits) demonstrates that the optimal mode of fast, beat-to-beat heart rate control by these nerves is by means of bursts of impulses arriving in every heart beat at well-timed moments. In vivo this is how the baroreflex stabilizes blood pressure at the expense of HRV.
[1] DeBoer RW, Karemaker JM, Strackee J. Hemodynamic fluctuations and baroreflex sensitivity in humans: a beat-to-beat model. Am J Physiol. 1987; 253: H680-H9.
[2] Karemaker JM, Wesseling KH. Variability in cardiovascular control: the baroreflex reconsidered. Cardiovascular Engineering. 2008; 8: 23-9.
[3] Karemaker JM. Counterpoint: Respiratory sinus arrhythmia is due to the baroreflex mechanism. Journal of applied physiology. 2009; 106: 1742-3.
[4] Billman GE. The effect of heart rate on the heart rate variability response to autonomic interventions. Frontiers in Physiology. 2013; 4: 222.
[5] Monfredi O, Lyashkov AE, Johnsen A-B, Inada S, Schneider H, Wang R, Nirmalan M, Wisloff U, Maltsev VA, Lakatta EG, Zhang H, Boyett MR. Biophysical characterization of the underappreciated and important relationship between heart rate variability and heart rate. Hypertension. 2014; 64: 1334-43.
[6] Yaniv Y, Tsutsui K, Lakatta E. Potential effects of intrinsic heart pacemaker cell mechanisms on dysrhythmic cardiac action potential firing. Frontiers in Physiology. 2015; 6.
[7] Armour JA. Potential clinical relevance of the ‘little brain' on the mammalian heart. Experimental Physiology. 2008; 93: 165-76.
[8] Karemaker J. Chaos in heart rate and the vagus nerve. A theoretical and experimental investigation. 3rd International workshop on computer analysis of blood pressure and heart rate sig-nals; 4-5 May 1995; Firenze, Italy1995.
[9] Karemaker JM. Vagal effects on heart rate: Different between up and down. Cardiovascular Oscillations (ESGCO), 2014 8th Conference of the European Study Group on; 2014 25-28 May 2014.
[10] Iriuchijima J, Kumada M. Activity of single vagal fibers efferent to the heart. The Japanese Journal of Physiology. 1964; 14: 479-87.
[11] Jewett DL. Activity of single efferent fibers in the cervical vagus nerve of the dog, with special reference to possible cardio-inhibitory fibres. The Journal of Physiology. 1964; 175: 321-57.
[12] Kunze DL. Reflex discharge patterns of cardiac vagal efferent fibres. J Physiol (London). 1972; 222: 1-15.
[13] Levy MN, Martin PJ. Neural regulation of the heartbeat. Ann Rev Physiol. 1981; 43: 443-53.
[14] Guevara MR, Glass L. Phase locking, period doubling bifurcations and chaos in a mathematical model of a periodically driven oscillator: A theory for the entrainment of biological oscillators and the generation of cardiac dysrhythmias. J Math Biol. 1982; 14: 1-23.
[15] Levy MN, Martin PJ, Iano T, Zieske H. Paradoxical effect of vagus nerve stimulation on heart rate in dogs. Circ Res. 1969; 25: 303-14.
[16] Michaels DC, Slenter V, A. J., Salata JJ, Jalife J. A model of dynamic vagus-sinoatrial node interactions. Am J Physiol. 1983; 245: H1043-H53.
[17] Abramovich-Sivan S, Akselrod S. A phase response curve based model: effect of vagal and sympathetic stimulation and interaction on a pacemaker cell. J Theo Biol. 1998; 192: 567-79.
[18] UFAW Staff. The UFAW handbook on the care and management of laboratory animals. 3rd edition. London and Edinburgh: E. & S. Livingstone; 1967.
[19] Karemaker JM, Borst C, Schreurs AW. Implantable stimulating electrode for baroreceptor afferent nerves in rabbits. Am J Physiol. 1980; 239: H706-9.
[20] Salata JJ, Jalife J. "Fade" of hyperpolarizing responses to vagal stimulation at the sinoatrial and atrioventricular nodes of the rabbit heart. Circ Res. 1985; 56: 718-27.
[21] Perlini S, Solda PL, Piepoli M, Sala-Gallini G, Calciati A, Finardi G, Bernardi L. Determinants of respiratory sinus arrhythmia in the vagotomized rabbit. Am J Physiol. 1995; 269: H909-15.
[22] Donders FC. Zur Physiologie des Nervus Vagus. Pflügers Archiv ges Physiologie. 1868; 1: 331-61.
[23] Brown GL, Eccles JC. The action of a single volley on the rhythm of the heart. J Physiol (London). 1934; 82: 211-41.
[24] Brown GL, Eccles JC. Further experiments on vagal inhibition of the heartbeat. J Physiol (London). 1934; 82: 242-57.
[25] Levy MN, Martin PJ, Iano T, Zieske H. Effects of single vagal stimuli on heart rate and atrioventricular conduction. Am J Physiol. 1970; 218: 1256-62.
[26] Stuesse SL, Levy MN, Zieske H. Phase-related sensitivity of the sinoatrial node to vagal stimuli in the isolated rat atrium. Circ Res. 1978; 43: 217-24.
[27] Levy MN, Wexberg S, Eckel C, Zieske H. The effect of changing interpulse intervals on the negative chronotropic response to repetitive bursts of vagal stimuli in the dog. Circ Res. 1978; 43: 570-6.
[28] Dong E, Reitz BA. Effect of timing of vagal stimulation on heart rate in the dog. Circ Res. 1970; 27: 635-46.
[29] Levy MN, Lano T, Zieske H. Effects of repetitive bursts of vagal activity on heart rate. Circ Res. 1972; 30: 186-95.
[30] Jalife J, Slenter VA, Salata JJ, Michaels DC. Dynamic vagal control of pacemaker activity in the mammalian sinoatrial node. Circ Res. 1983; 52: 642-56.
[31] Peňáz J. Frequency response of the cardiac chronotropic action of the vagus in the rabbit. Arch Physiol Biochem. 1962; 70: 636-50.
[32] Berger RD, Saul JP, Cohen RJ. Transfer function analysis of autonomic regulation. I. Canine atrial rate response. Am J Physiol. 1989; 256: H142-52.
[33] Saul JP, Berger RD, Chen MH, Cohen RJ. Transfer function analysis of autonomic regulation. II. Respiratory sinus arrhythmia. Am J Physiol. 1989; 256: H153-61.
[34] Saul JP. Transfer function analysis of cardiorespiratory variability to assess autonomic regulation. Clin Sci (Colch). 1996; 91 Suppl: 101.
[35] Mokrane A, LeBlanc AR, Nadeau R. Transfer function analysis of vagal control of heart rate during synchronized vagal stimulation. Am J Physiol. 1995; 269: H1931-40.
[36] Richman JS, Moorman JR. Physiological time-series analysis using approximate entropy and sample entropy. Am J Physiol Heart Circ Physiol. 2000; 278: H2039-49.
[37] Costa M, Goldberger AL, Peng CK. Multiscale Entropy Analysis of Complex Physiologic Time Series. Phys Rev Lett. 2002; 89: 068102.
[38] de Boer RW, Karemaker JM, Strackee J. Description of heart-rate variability data in accordance with a physiological model for the genesis of heartbeats. Psychophysiology. 1985; 22: 147-55.
[39] Berger RD, Akselrod S, Gordon D, Cohen RJ. An efficient algorithm for spectral analysis of heart rate variability. IEEE Trans Biomed Eng. 1986; 33: 900-4.
[40] Camm AJ, Malik M, Bigger JT, Breithardt G, Cerutti S, Cohen RJ, et al. Heart Rate Variability - Standards of Measurement, Physiological Interpretation, and Clinical Use [Review]. Circulation. 1996; 93: 1043-65.
[41] Slenter VA, Salata JJ, Jalife J. Vagal control of pacemaker periodicity and intranodal conduction in the rabbit sinoatrial node. Circ Res. 1984; 54: 436-46.
[42] McAllen RM, Salo LM, Paton JFR, Pickering AE. Processing of central and reflex vagal drives by rat cardiac ganglion neurones: an intracellular analysis. J Physiol. 2011; 589: 5801-18.
[43] McAllen RM, Spyer KM. The baroreceptor input to cardiac vagal motoneurones. J Physiol. 1978; 282: 365.
[44] Karemaker JM. Vagal effects of the baroreflex on heart rate [PhD thesis]. Amsterdam: University of Amsterdam; 1980.
[45] Borst C, Karemaker JM. Time delays in the human baroreceptor reflex. J Auton Nerv Syst. 1983; 9: 399-409.
[46] Seidel H, Herzel H, Eckberg DL. Phase dependencies of the human baroreceptor reflex. Am J Physiol Heart Circ Physiol. 1997; 41: H2040-H53.
[47] Pickering TG, Davies J. Estimation of the conduction time of the baroreceptor-cardiac reflex in man. Cardiovasc Res. 1973; 7: 213-9.
[48] Karemaker JM, Borst C. Measurement of baroreflex sensitivity in hypertension research. In: Sleight P (editor): Arterial baroreceptors and hypertension. Oxford University Press: Oxford; 1980. p. 455-61.
[49] Smyth HS, Sleight P, Pickering GW. Reflex regulation of arterial pressure during sleep in man. A quantitative method of assessing baroreflex sensitivity. Circ Res. 1969; 24: 109-21.
[50] Westerhof BE, Gisolf J, Stok WJ, Wesseling KH, Karemaker JM. Time-domain cross-correlation baroreflex sensitivity: performance on the EUROBAVAR data set. J Hypertens. 2004; 22: 1371-80.
[51] Force T. Heart rate variability. Standards of measurement, physiological interpretation, and clinical use. Task force of the European society of cardiology and the North American society of pacing and electrophysiology. Eur Heart J. 1996; 17: 354-81.
[52] Golenhofen K, Lippross H. Mechanishce Koppelungswirkungen der Atmung auf den Herzschlag [Mechanical coupling effects between respiration and heart rhythm]. [German]. Pflügers Arch. 1969; 309: 159-66.
[53] Bolter CPWSJ. Influence of right atrial pressure on the cardiac pacemaker response to vagal stimulation. Am J Physiol Regul Integr Comp Physiol. 1999; 276: R1112-R7.
[54] Purves RD. Muscarinic excitation: a microelectrophoretic study on cultured smooth muscle cells. Br J Pharmacol. 1974; 52: 77-86.
[55] Purves RD. Function of muscarinic and nicotinic acetylcholine receptors. Nature. 1976; 261(13 May 1976): 149-51.
[56] Bouman LN, Gerlings ED, Biersteker PA, Bonke FIM. Pacemaker shift in the sino-atrial node during vagal stimulation. Pfluegers Arch. 1968; 302: 255-67.
[57] Jalife J, Moe GK. Phasic effects of vagal stimulation on pacemaker activity of the isolated sinus node of the young cat. Circ Res. 1979; 45: 595-608.
[58] Demir SS, Clark JW, Giles WR. Parasympathetic modulation of sinoatrial node pacemaker activity in rabbit heart: a unifying model. Am J Physiol Heart Circ Physiol. 1999; 276: H2221-44.
[59] Gilbey MP, Jordan D, Richter DW, Spyer KM. Synaptic mechanisms involved in the inspiratory modulation of vagal cardio-inhibitory neurones in the cat. J Physiol. 1984; 356: 65-78.
[60] Parker P, Celler BG, Potter EK, McCloskey DI. Vagal stimulation and cardiac slowing. J Auton Nerv Syst. 1984; 11: 226-31.
[61] Faes TJ, De Neeling NN, Kingma R, TenVoorde BJ, Karemaker JM. On the quantification of heart rate changes in autonomic function tests: relations between measures in beats per minute, seconds and dimensionless ratios. Clin Sci (Colch). 1995; 89: 557-64.
[62] Yaniv Y, Lyashkov AE, Lakatta EG. Impaired signaling intrinsic to sinoatrial node pacemaker cells affects heart rate variability during cardiac disease. J Clin Trials. 2013; 4: 152.
[63] Jongsma HJ, Tsjernina L, de Bruijne J. The establishment of regular beating in populations of pacemaker heart cells. A study with tissue-cultured rat heart cells. J Mol Cell Cardiol. 1983; 15: 123-33.
[64] Lu H-H, Lange G, McC. Brooks C. Factors Controlling Pacemaker Action in Cells of the Sinoatrial Node. Circ Res. 1965; 17: 460-71.
[65] Bouman LN, Jongsma HJ. Structure and function of the sino-atrial node: a review. Eur Heart J. 1986; 7: 94-104.
[66] Hill L, Barnard H. The Influence of the Force of Gravity on the Circulation Part II. Section I. The action of the respiratory pump. Section II. The escape of the heart from vagal arrest. Section III. The mean pressure of the vascular system. J Physiol. 1897; 21: 323-52.
[67] Wallace AG, Daggett WM. Pacemaker activity during vagal escape rhythms. Circ Res. 1964; 15: 93-102.
[68] Donlon JV Jr. Anesthesia for ophthalmologic surgery. ASA Refresher Courses in Anesthesiology. 1988; 16: 81-92.