Plasmodium falciparum histoaspartic protease inhibitor: Toxicity investigation and docking study of 2-(2-benzoyl-4-methylphenoxy) quinoline-3-carbaldehyde derivatives
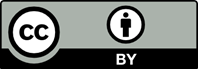
Aspartic proteases can hydrolyze peptide bonds, making them potential targets for drug development against malaria parasites. In particular, inhibiting the histoaspartic protease (HAP) can disrupt the growth phase of Plasmodium falciparum and its ability to degrade hemoglobin for protein synthesis. Compound 5, specifically designed as 2-(2-benzoyl-4-methylphenoxy)quinoline-3-carbaldehyde, served as the basis for designing 50 hypothetical compounds (A1-A50). These compounds were subjected to in silico screening to assess their toxicity profiles, pharmacokinetics, bioactivity scores, and theoretical binding affinities, as a part of the drug design protocol. Out of the 50 compounds, nine lead candidates showed no toxicity to human cells. In addition, ten standard reference antimalarial drugs were included in this study for comparison. The highest binding energies were observed for compound A5 (−11.2 kcal/mol) and A31 (−11.3 kcal/mol), surpassing the performance of mefloquine, the best reference drug, which ranked ninth with a binding energy of (−9.6 kcal/mol). Compound A31 did not exhibit the evidence of interaction with either Asp215 or His32, whereas compound A5 displayed π-π stacking interactions with His32. Mefloquine also did not show any interaction with Asp215 or His32. Moreover, compound A5 demonstrated greater hydrophobic interactions at the active site with most binding residues, except for Lys7 in the hydrophobic region. This characteristic suggests that compound A5 may have the ability to adopt a smaller surface area, exhibit increased biological activity, and have reduced interactions with water, which could facilitate slower clearance. Based on the assessment of various drug-likeness parameters, compound A5 (2-(2-benzoyl-4-methylphenoxy)-7-methylquinoline-3-carbaldehyde) is a potential lead candidate for the development of a new antimalarial drug.
- World Health Organization, 2022, WHO Guidelines for Malaria. WHO/UCN/GMP/2022.01 Rev. 2. Geneva: World Health Organization.
- Jensen AR, Adams Y, Hviid L, 2020, Cerebral Plasmodium falciparum malaria: The role of PfEMP1 in its pathogenesis and immunity, and PfEMP1‐based vaccines to prevent it. Immunol. Rev., 293: 230–252. https://doi.org/10.1111/imr.12807
- Karthik L, Kumar G, Keswani T, et al., 2014, Protease inhibitors from marine actinobacteria as a potential source for antimalarial compound. PLoS One, 9: e90972.
- Francis SE, Banerjee R, Goldberg DE, 1997, Biosynthesis and maturation of the malaria aspartic hemoglobinases plasmepsins I and II. J Biol Chem, 272: 14961–14968. https://doi.org/10.1074/jbc.272.23.14961
- Nasamu AS, Polino AJ, Istvan ES, 2020, Malaria parasite plasmepsins: More than just plain old degradative pepsins. J Biol Chem, 295: 8425–8441. https://doi.org/10.1074/jbc.REV120.009309
- Boddey JA, Hodder AN, Günther S, et al., 2010, An aspartyl protease directs malaria effector proteins to the host cell. Nature, 463: 627–631. https://doi.org/10.1038/nature08728
- Roy KK, 2017, Targeting the active sites of malarial proteases for antimalarial drug discovery: Approaches, progress and challenges. Int J Antimicrob Agents, 50: 287–302. https://doi.org/10.1016/j.ijantimicag.2017.04.006
- Zhou W, Wang H, Yang Y, et al., 2020, Chloroquine against malaria, cancers and viral diseases. Drug Discov Today, 25: 2012–2022. https://doi.org/10.1016/j.drudis.2020.09.010
- Hong SH, Ismail IA, Kang SM, et al., 2016, Cinnamaldehydes in cancer chemotherapy. Phytother Res, 30: 754–767. https://doi.org/10.1002/ptr.5592
- Nair A, Amalraj A, Jacob J, et al., 2019, Non-curcuminoids from turmeric and their potential in cancer therapy and anticancer drug delivery formulations. Biomolecules, 9: 13. https://doi.org/10.3390/biom9010013
- Ma HS, Wang EL, Xu WF, et al., 2018, Overexpression of DNA (cytosine-5)-methyltransferase 1 (DNMT1) and DNA (cytosine-5)-methyltransferase 3A (DNMT3A) is associated with aggressive behavior and hypermethylation of tumor suppressor genes in human pituitary adenomas. Med Sci Monit, 24: 4841–4850. https://doi.org/10.12659/MSM.910608
- Schneidman‐Duhovny D, Inbar Y, Nussinov R, et al., 2005, Geometry‐based flexible and symmetric protein docking. Proteins, 60: 224–231. https://doi.org/10.1002/prot.20562
- Banerjee R, Liu J, Beatty W, et al., 2002, Four plasmepsins are active in the Plasmodium falciparum food vacuole, including a protease with an active-site histidine. Proc Natl Acad Sci, 99: 990–995. https://doi.org/10.1073/pnas.022630099
- Bhaumik P, Gustchina A, Wlodawer A, 2012, Structural studies of vacuolar plasmepsins. Biochim Biophys Acta, 1824: 207–223. https://doi.org/10.1016/j.bbapap.2011.04.008
- Available from: https://tox-new.charite.de [Last accessed on 2023 May 31].
- Available from: https://pubchem.ncbi.nlm.nih.gov [Last accessed on 2023 May 25].
- Lipinski B, Herzog H, Kops ER, et al., 1997 Expectation maximization reconstruction of positron emission tomography images using anatomical magnetic resonance information. IEEE Trans Med Imaging, 16: 129–136. https://doi.org/10.1109/42.563658
- Available from: https://www.rcsb.org/ [Last accessed on 2023 Apr 20].
- Available from: https://lmmd.ecust.edu.cn/admetsar2 [Last accessed on 2023 Mar 15].
- Yang H, Sun L, Li W, et al., 2018, In silico prediction of chemical toxicity for drug design using machine learning methods and structural alerts. Front Chem, 6: 30. https://doi.org/10.3389/fchem.2018.00030
- Trott O, Olson AJ, 2010, AutoDock Vina: Improving the speed and accuracy of docking with a new scoring function, efficient optimization, and multithreading. J Comput Chem, 31: 455. https://doi.org/10.1002/jcc.21334
- Jacobs AC, Hatfield KP, 2013. History of chronic toxicity and animal carcinogenicity studies for pharmaceuticals. Vet Pathol, 50: 324-333. https://doi.org/10.1177/0300985812450727
- Daina A, Olivier M, Vincent Z, 2017, SwissADME: A free web tool to evaluate pharmacokinetics, drug-likeness and medicinal chemistry friendliness of small molecules. Sci Rep, 7: 42717. https://doi.org/10.1038/srep42717
- Klopman G, Stefan LR, Saiakhov RD, 2002, ADME evaluation: 2. A computer model for the prediction of intestinal absorption in humans. Eur J Pharm Sci, 17: 253–263. https://doi.org/10.1016/s0928-0987(02)00219-1
- Lipinski CA, Lombardo F, Dominy BW, et al., 2012, Experimental and computational approaches to estimate solubility and permeability in drug discovery and development settings. Adv Drug Deliv Rev, 64: 4–17. https://doi.org/10.1016/j.addr.2012.09.019
- Huang H, Rong H, Li X, et al., 2008, The crystal structure and identification of NQM1/YGR043C, a transaldolase from Saccharomyces cerevisiae. Proteins, 73: 1076–1081. https://doi.org/10.1002/prot.22237
- Wang B, Yang, LP, Zhang XZ, et al., 2009, New insights into the structural characteristics and functional relevance of the human cytochrome P450 2D6 enzyme. Drug Metab Rev, 41: 573–643. https://doi.org/10.1080/03602530903118729
- Kakinuma T, Hwang, ST, 2006, Chemokines, chemokine receptors, and cancer metastasis. J. Leukoc Biol, 79: 639–651. https://doi.org/10.1189/jlb.1105633
- McEwan IJ, 2009, Nuclear receptors: One big family. Methods Mol Biol, 505: 3–18. https://doi.org/10.1007/978-1-60327-575-0_1
- Schwab A, Fabian A, Hanley PJ, et al., 2012, Role of ion channels and transporters in cell migration. Physiol Rev, 92: 1865–1913. https://doi.org/10.1152/physrev.00018.2011
- Copeland RA, 2013, Evaluation of Enzyme Inhibitors in Drug Discovery: A Guide for Medicinal Chemists and Pharmacologists. United States: John Wiley & Sons.
- Leung D, Abbenante G, Fairlie DP, 2000, Protease inhibitors: Current status and future prospects. J Med Chem, 43: 305–341. https://doi.org/10.1021/jm990412m
- Barker BS, Young GT, Soubrane CH, et al., 2017, Ion channels. In: Conn’s Translational Neuroscience. 1st ed. London: Elsevier Academic Press, p11–43. https://doi.org/10.1016/B978-0-12-802381-5.00002-6
- Ma FH, Li C, Liu YL, 2020, Mimicking molecular chaperones to regulate protein folding. Adv Mater, 32: e1805945. https://doi.org/10.1002/adma.201805945
- Savojardo C, Manfredi M, Martelli PL, et al., 2021, Solvent accessibility of residues undergoing pathogenic variations in humans: From protein structures to protein sequences. Front Mol Biosci, 7: 626363. https://doi.org/10.3389/fmolb.2020.626363
- Gromiha MM, Ahmad S, 2005, Role of solvent accessibility in structure based drug design. Curr Comput Aided Drug Des, 1: 223–235.