Hydrolytic Expansion Induces Corrosion Propagation for Increased Fe Biodegradation
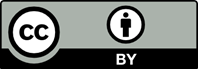
Fe is regarded as a promising bone implant material due to inherent degradability and high mechanical strength, but its degradation rate is too slow to match the healing rate of bone. In this work, hydrolytic expansion was cleverly exploited to accelerate Fe degradation. Concretely, hydrolyzable Mg2 Si was incorporated into Fe matrix through selective laser melting and readily hydrolyzed in a physiological environment, thereby exposing more surface area of Fe matrix to the solution. Moreover, the gaseous hydrolytic products of Mg2 Si acted as an expanding agent and cracked the dense degradation product layers of Fe matrix, which offered rapid access for solution invasion and corrosion propagation toward the interior of Fe matrix. This resulted in the breakdown of protective degradation product layers and even the direct peeling off of Fe matrix. Consequently, the degradation rate for Fe/Mg2 Si composites (0.33 mm/y) was significantly improved in comparison with that of Fe (0.12 mm/y). Meanwhile, Fe/Mg2 Si composites were found to enable the growth and proliferation of MG-63 cells, showing good cytocompatibility. This study indicated that hydrolytic expansion may be an effective strategy to accelerate the degradation of Fe-based implants.
1. Gao C, Yao M, Li S, et al., 2019, Highly Biodegradable and Bioactive Fe-Pd-Bredigite Biocomposites Prepared by Selective Laser Melting. J Adv Res, 20:91–104. DOI:10.1016/j.jare.2019.06.001.
2. Shuai C, Li S, Peng S, et al., 2019, Biodegradable Metallic Bone Implants. Mater Chem Front, 3(1):544–62. DOI: 10.1039/C8QM00507A.
3. Yang YY, He C, Dianyu E, et al., 2019, Mg Bone Implant: Features, Developments and Perspectives. Mater Design, 185:108259. DOI: 10.1016/j.matdes.2019.108259.
4. Gao C, Yao M, Shuai C, et al., 2019, Nano-SiC Reinforced Zn Biocomposites Prepared Via Laser Melting: Microstructure, Mechanical Properties and Biodegradability. J Mater Sci Tech, 35:2608–17. DOI: 10.1016/j.jmst.2019.06.010.
5. Shuai C, Yang W, He C, et al., 2019, A Magnetic Micro-Environment in Scaffolds for Stimulating Bone Regeneration. Mater Des, 185:108275. DOI: 10.1016/j.matdes.2019.108275.
6. Wang G, Qi F, Yang W, et al., 2019, Crystallinity and Reinforcement in Poly-L-Lactic Acid Scaffold Induced by Carbon Nanotubes. Advances in Polymer Technology, 2019:10. DOI:10.1155/2019/8625325.
7. He S, Yang S, Zhang Y, et al., 2019, LncRNA ODIR1 Inhibits Osteogenic Differentiation of hUC-MSCs Through the FBXO25/H2BK120ub/H3K4me3/OSX axis. Cell Death Dis, 10:1–16. DOI: 10.1038/s41419-019-2148-2.
8. Zivic F, Grujovic N, Pellicer E, et al., 2018, Biodegradable Metals as Biomaterials for Clinical Practice: Iron-Based Materials, Biomaterials in Clinical Practice. Springer, Berlin.pp. 225–80. DOI: 10.1007/978-3-319-68025-5_9.
9. Deng Y, Yang Y, Gao C, et al., 2018, Mechanism for Corrosion Protection of β-TCP Reinforced ZK60 Via Laser Rapid Solidification. Int J Bioprint, 4(1):27–41. DOI: 10.18063/IJB.v4i1.124.
10. Hermawan H, Dubé D, Mantovani D, 2007, Development of Degradable Fe-35Mn Alloy for Biomedical Application. Adv Mater Res, 15:107–12. DOI: 10.4028/www.scientific.net/AMR.15-17.107.
11. Schinhammer M, Steiger P, Moszner F, et al. 2013, Degradation Performance of Biodegradable FeMnC (Pd) Alloys. Mater Sci Eng C, 33:1882–93. DOI: 10.1016/j.msec.2012.10.013.
12. Zhou J, Yang Y, Alonso Frank M, et al., 2016, Accelerated Degradation Behavior and Cytocompatibility of Pure Iron Treated with Sandblasting. ACS Appl Mater Interfaces, 8:26482–92. DOI: 10.1021/acsami.6b07068.
13. Moravej M, Purnama A, Fiset M, et al., 2010, Electroformed Pure Iron as a New Biomaterial for Degradable Stents: In Vitro Degradation and Preliminary Cell Viability Studies. Acta Biomater, 6:1843–51. DOI: 10.1016/j.actbio.2010.01.008.
14. Jiang W, Wang J, Yu W, et al., 2019, In Situ Formation of a Gradient Mg2Si/Mg Composite with Good Biocompatibility. Surf Coat Technol, 361:255–62. DOI: 10.1016/j.surfcoat.2018.12.107.
15. Sikora-Jasinska M, Paternoster C, Mostaed E, et al., 2017, Synthesis, Mechanical Properties and Corrosion Behavior of Powder Metallurgy Processed Fe/Mg2Si Composites for Biodegradable Implant Applications. Mater Sci Eng C, 81(2):511–21. DOI: 10.1016/j.msec.2017.07.049.
16. Lee JY, An J, Chua CK, 2017, Fundamentals and Applications of 3D Printing for Novel Materials. Appl Mater Today, 7:120–33. DOI: 10.1016/j.apmt.2017.02.004.
17. An J, Teoh JEM, Suntornnond R, et al., 2015, Design and 3D Printing of Scaffolds and Tissues. Engineering, 1:261–68. DOI: 10.15302/J-ENG-2015061.
18. Sing SL, Huang S, Yeong WY, 2020, Effect of Solution Heat Treatment on Microstructure and Mechanical Properties of Laser Powder Bed Fusion Produced Cobalt-28chromium-6molybdenum. Mater Sci Eng A, 769:138511. DOI: 10.1016/j.msea.2019.138511.
19. Nasab MH, Giussani A, Gastaldi D, et al., 2019, Effect of Surface and Subsurface Defects on Fatigue Behavior of AlSi10Mg Alloy Processed by Laser Powder Bed Fusion (L-PBF). Metals, 9:1063. DOI: 10.3390/met9101063.
20. Yu W, Sing S, Chua C, et al., 2019, Particle-Reinforced Metal Matrix Nanocomposites Fabricated by Selective Laser Melting: A State of the Art Review. Prog Mater Sci, 104:330‑379. DOI: 10.1016/j.pmatsci.2019.04.006.
21. Dadbakhsh S, Mertens R, Hao L, et al., 2019, Selective Laser Melting to Manufacture “In Situ” Metal Matrix Composites: A Review. Adv Eng Mater, 21:1801244. DOI:10.1002/adem.201801244.
22. Hariharan K, Arumaikkannu G, 2016, Structural, Mechanical and In Vitro Studies on Pulsed Laser Deposition of Hydroxyapatite on Additive Manufactured Polyamide Substrate. Int J Bioprint, 2:85–94. DOI: 10.1016/S0021-9290(06)83793-0.
23. Lepowsky E, Tasoglu S, 2018, 3D Printing for Drug Manufacturing: A Perspective on the Future of Pharmaceuticals. Int J Bioprint, 4:119. DOI: 10.18063/IJB. v4i1.119.
24. Yap CY, Chua CK, Dong ZL, et al., 2015, Review of Selective Laser Melting: Materials and Applications. Appl Phys Rev, 2:041101. DOI: 10.1063/1.4935926.
25. Loh LE, Chua CK, Yeong WY, et al., 2015, Numerical Investigation and an Effective Modelling on the Selective Laser Melting (SLM) Process with Aluminium Alloy 6061. Int J Heat Mass Transfer, 80:288–300. DOI: 10.1016/j. ijheatmasstransfer.2014.09.014.
26. Sun Z, Tan X, Tor SB, et al. 2018, Simultaneously Enhanced Strength and Ductility for 3D-Printed Stainless Steel 316L by Selective Laser Melting. NPG Asia Materials, 10:127. DOI:10.1038/s41427-018-0018-5.
27. Li Y, Zhou K, Tan P, et al., 2018, Modeling Temperature and Residual Stress Fields in Selective Laser Melting. Int J Mech Sci, 136:24–35. DOI: 10.1016/j.ijmecsci.2017.12.001.
28. Yu W, Sing SL, Chua CK, et al., 2019, Influence of Re-Melting on Surface Roughness and Porosity of AlSi10Mg Parts Fabricated by Selective Laser Melting. J Alloys Compd,792:574–81. DOI: 10.1016/j.jallcom.2019.04.017.
29. Kuo C, Chua C, Peng P, et al., 2019, Microstructure Evolution and Mechanical Property Response Via 3D Printing Parameter Development of Al-Sc alloy. Virtual Phys Prototyp, 15:1–10. DOI: 10.1080/17452759.2019.1698967.
30. Vanarase AU, Muzzio FJ, 2011, Effect of Operating Conditions and Design Parameters in a Continuous Powder Mixer. Powder Technol, 208:26–36. DOI: 10.1016/j.powtec.2010.11.038.
31. Portillo PM, 2008, Quality by Design for Continuous Powder Mixing. Rutgers University-Graduate School, New Brunswick.
32. Shuai C, Zan J, Yang Y, et al., 2019, Surface Modification Enhances Interfacial Bonding in PLLA/MgO Bone Scaffold. Mater Sci Eng C, 108:110486. DOI: 10.1016/j.msec.2019.110486.
33. Shuai C, Xu Y, Feng P, et al., 2019, Antibacterial Polymer Scaffold Based on Mesoporous Bioactive Glass Loaded with In Situ Grown Silver. Chem Eng J, 374:304–15. DOI: 10.1016/j.cej.2019.03.273.
34. Wang S, Xu Y, Zhou J, et al., 2017, In Vitro Degradation and Surface Bioactivity of Iron-Matrix Composites Containing Silicate-Based Bioceramic. Bioact Mater, 2:10–18. DOI: 10.1016/j.bioactmat.2016.12.001.
35. Shuai C, Li S, Wang G, et al., 2019, Strong Corrosion Induced by Carbon Nanotubes to Accelerate Fe Biodegradation. Mater Sci Eng C, 104(5):109935. DOI: 10.1016/j.msec.2019.109935.
36. Wang H, Zheng Y, Li Y, et al., 2017, Improvement of In Vitro Corrosion and Cytocompatibility of Biodegradable Fe Surface Modified by Zn Ion Implantation. Appl Surf Sci, 403(12):168–76. DOI: 10.1016/j.apsusc.2017.01.158.
37. Shuai C, Cheng Y, Yang Y, et al., 2019, Laser Additive Manufacturing of Zn-2Al Part for Bone Repair: Formability, Microstructure and Properties. J Alloys Comp, 798:606–15. DOI: 10.1016/j.jallcom.2019.05.278.
38. Cheng J, Liu B, Wu Y, et al., 2013, Comparative In Vitro Study on Pure Metals (Fe, Mn, Mg, Zn and W) as Biodegradable Metals. J Mater Sci Tech, 29:619–27. DOI: 10.1016/j. jmst.2013.03.019.
39. Shuai C, Liu G, Yang Y, et al., 2019, Functionalized BaTiO3 Enhances Piezoelectric Effect Towards Cell Response of Bone Scaffold. Colloids Surf, 3:110587–94. DOI: 10.1016/j.colsurfb.2019.110587.
40. Feng P, Kong Y, Yu L, et al., 2019, Molybdenum Disulfide Nanosheets Embedded with Nanodiamond Particles: Co-Dispersion Nanostructures as Reinforcements for Polymer Scaffolds. Appl Mater Today, 17:216–26. DOI: 10.1016/j.apmt.2019.08.005.
41. Prakasam M, Locs J, Salma-Ancane K, et al., 2017, Biodegradable Materials and Metallic Implants a Review.J Funct Biomater, 8:44. DOI: 10.3390/jfb8040044.
42. Gao C, Peng S, Feng P, et al., 2017, Bone Biomaterials and Interactions with Stem Cells. Bone Res, 5:17059. DOI:10.1038/boneres.2017.59.
43. Dehestani M, Adolfsson E, Stanciu LA, 2016, Mechanical Properties and Corrosion Behavior of Powder Metallurgy Iron-Hydroxyapatite Composites for Biodegradable Implant Applications. Mater Des, 109:556–69. DOI: 10.1016/j.matdes.2016.07.092.
44. Kato K, Ochiai S, Yamamoto A, et al., 2013, Novel Multilayer Ti Foam With Cortical Bone Strength and Cytocompatibility. Acta Biomater, 9:5802–09. DOI: 10.1016/j.actbio.2012.11.018.
45. Panemangalore DB, Shabadi R, Tingaud D, et al., 2019, Biocompatible Silica-Based Magnesium Composites. J Alloys Comp, 772(15):49–57. DOI: 10.1016/j.jallcom.2018.09.060.
46. Zhang Y, Wang L, Sun W, et al., 2019, Membrane Technologies for Li+/Mg2+ Separation From Salt-LakeBrines and Seawater: A Comprehensive Review. J Ind Eng Chem, 81:7–23. DOI: 10.1016/j.jiec.2019.09.002.
47. Bowen PK, Shearier ER, Zhao S, et al., 2016, Biodegradable Metals for Cardiovascular Stents: From Clinical Concerns to Recent Zn-Alloys. Adv Healthc Mater, 5:1121–40. DOI:10.1002/adhm.201501019.
48. Shuai C, Yang Y, Feng P, et al., 2018, A Multi-Scale Porous Scaffold Fabricated by a Combined Additive Manufacturing and Chemical Etching Process for Bone Tissue Engineering. Int J Bioprint, 4:133. DOI: 10.18063/IJB.v4i2.133.
49. Gorejová R, Haverová L, Oriňaková R, et al., 2019, Recent Advancements in Fe-Based Biodegradable Materials for Bone Repair. J M Sci, 54(12):1913–47. DOI: 10.1007/s10853-018-3011-z.
50. Jin J, Tan Y, Liu R, et al., 2018, Synergy Effect of Attapulgite, Rubber, and Diatomite on Organic Montmorillonite-Modified Asphalt. J Mater Civil Eng, 31:04018388. DOI: 10.1061/(ASCE)MT.1943-5533.0002601.
51. Zhao Y, Jamesh MI, Li WK, et al., 2014, Enhanced Antimicrobial Properties, Cytocompatibility, and Corrosion Resistance of Plasma-Modified Biodegradable Magnesium Alloys. Acta Biomater, 10:544–56. DOI: 10.1016/j.actbio.2013.10.012.