Patterning of tissue spheroids biofabricated from human fibroblasts on the surface of electrospun polyurethane matrix using 3D bioprinter
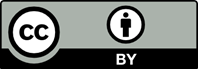
Organ printing is a computer-aided additive biofabrication of functional three-dimensional human tissue and organ constructs according to digital model using the tissue spheroids as building blocks. The fundamental biological principle of organ printing technology is a phenomenon of tissue fusion. Closely placed tissue spheroids undergo tissue fusion driven by surface tension forces. In order to ensure tissue fusion in the course of post-printing, tissue spheroids must be placed and maintained close to each other. We report here that tissue spheroids biofabricated from primary human fibroblasts could be placed and maintained on the surface of biocompatible electrospun polyurethane matrix using 3D bioprinter according to desirable pattern. The patterned tissue spheroids attach to polyurethane matrix during several hours and became completely spread during several days. Tissue constructions biofabricated by spreading of patterned tissue spheroids on the biocompatible electrospun polyurethane matrix is a novel technological platform for 3D bioprinting of human tissue and organs.
1. Derby B, 2012, Printing and prototyping of tissues and scaffolds. Science, vol.338(6109): 921–926. http://dx.doi.org/10.1126/science.1226340
2. Murphy S V and Atala A, 2014, 3D bioprinting of tissues and organs. Nature Biotechnology, vol.32(8): 773–785. http://dx.doi.org/10.1038/nbt.2958
3. Atala A and Yoo J J, 2015, Essentials of 3D Biofabrication and Translation, Academic Press, United States.
4. Chua C K and Yeong W Y, 2015, Bioprinting: Principles and Applications, World Scientific Publishing Company, Singapore.
5. Gao G and Cui X, 2015, Three-dimensional bioprinting in tissue engineering and regenerative medicine. Biotechnology Letters, vol.37(12): 1–9. http://dx.doi.org/10.1007/s10529-015-1975-1
6. Ozbolat IT, 2015, Bioprinting scale-up tissue and organ constructs for transplantation. Trends in Biotechnology, vol.33(7): 395–400. http://dx.doi.org/10.1016/j.tibtech.2015.04.005
7. Mironov V, Kasyanov V, Drake C, et al., 2008, Organ printing: Promises and challenges. Regenerative Medicine, vol.3(1): 93–103. http://dx.doi.org/10.2217/17460751.3.1.93
8. Mironov V, Visconti R P, Kasyanov V, et al., 2009, Organ printing: Tissue spheroids as building blocks. Biomaterials, vol.30(12): 2164–2174. http://dx.doi.org/10.1016/j.biomaterials.2008.12.084
9. Perez-Pomares J M and Foty R A, 2006, Tissue fusion and cell sorting in embryonic development and disease: Biomedical implications. BioEssays, vol.28(8): 809–821. http://dx.doi.org/10.1002/bies.20442
10. Hajdu Z, Mironov V, Mehesz A N, et al., 2010, Tissue spheroid fusion-based in vitro screening assays for analysis of tissue maturation. Journal of Tissue Engineering and Regenerative Medicine, vol.4(8): 659–664. http://dx.doi.org/10.1002/term.291
11. Huang G S, Tseng C S, Linju Yen B, et al., 2013, Solid freeform-fabricated scaffolds designed to carry multicellular mesenchymal stem cell spheroids for cartilage re-generation. European Cells and Materials, vol.26: 179– 194; discussion 194.
12. Ozbolat IT and Yu Y, 2013, Bioprinting toward organ fabrication: Challenges and future trends. IEEE Transactions on Biomedical Engineering, vol.60(3): 691–699. http://dx.doi.org/10.1109/TBME.2013.2243912
13. Schon B S, Schrobback K, van der Ven M, et al., 2012, Validation of a high-throughput microtissue fabrication process for 3D assembly of tissue engineered cartilage constructs. Cell and Tissue Research, vol.347(3): 629–642. http://dx.doi.org/10.1007/s00441-011-1311-6
14. Jakab K, Neagu A, Mironov V, et al., 2004, Engineering biological structures of prescribed shape using self-assembling multicellular systems. Proceeding of the National Academy of Science of the United States of America, vol.101(9): 2864–2869. http://dx.doi.org/10.1073/pnas.0400164101
15. Skardal A and Atala A, 2015, Biomaterials for integration with 3-D bioprinting. Annals of Biomedical Engineering, vol.43(3): 730–746. http://dx.doi.org/10.1007/s10439-014-1207-1
16. Itoh M, Nakayama K, Noguchi R, et al., 2015, Scaffold-free tubular tissues created by a bio-3D printer undergo remodeling and endothelialization when implanted in rat aortae. PloS ONE, vol.10(9): e0136681. http://dx.doi.org/10.1371/journal.pone.0136681
17. Dvir T, Timko B P, Kohane D S, et al., 2011, Nanotechnological strategies for engineering complex tissues. Nature Nanotechnology, vol.6(1): 13–22. http://dx.doi.org/10.1038/nnano.2010.246
18. Rezende R A, Azevedo F S, Pereira F D A S, et al., 2012, Nanotechnological strategies for biofabrication of human organs. Journal of Nanotechnology, vol.2012: 1–10. http://dx.doi.org/10.1155/2012/149264
19. Mironov V, Kasyanov V and Markwald R R, 2008, Nanotechnology in vascular tissue engineering: From nanoscaffolding towards rapid vessel biofabrication. Trends in Biotechnology, vol.26(6): 338–344. http://dx.doi.org/10.1016/j.tibtech.2008.03.001
20. Pham Q P, Sharma U and Mikos A G, 2006, Electrospinning of polymeric nanofibers for tissue engineering applications: A review. Tissue Engineering, vol.12(5): 1197–1211. http://dx.doi.org/10.1089/ten.2006.12.1197
21. Beachley V, Kasyanov V, Nagy-Mehesz A, et al., 2014, The fusion of tissue spheroids attached to pre-stretched electrospun polyurethane scaffolds. Journal of Tissue En-gineering, vol.5: 1–11. http://dx.doi.org/10.1177/2041731414556561
22. Chua K N, Lim W S, Zhang P, et al., 2005, Stable immobilization of rat hepatocyte spheroids on galactosylated nanofiber scaffold. Biomaterials, vol.26(15): 2537–2547. http://dx.doi.org/10.1016/j.biomaterials.2004.07.040
23. Lee H J, Lee S J, Uthaman S, et al., 2015, Biomedical
applications of magnetically functionalized organic/inorganic hybrid nanofibers. International Journal of Molecular Sciences, vol.16(6): 13661–13677. http://dx.doi.org/10.3390/ijms160613661
24. Ho V H, Muller K H, Barcza A, et al., 2010, Generation and manipulation of magnetic multicellular spheroids. Biomaterials, vol.31(11): 3095–3102. http://dx.doi.org/10.1016/j.biomaterials.2009.12.047
25. Lin R Z, Chu W C, Chiang C C, et al., 2008, Magnetic reconstruction of three-dimensional tissues from multi-cellular spheroid. Tissue Engineering Part C: Methods, vol.14(3): 197–205. http://dx.doi.org/10.1089/ten.tec.2008.0061
26. Mattix B, Olsen T R, Gu Y, et al., 2014, Biological magnetic cellular spheroids as building blocks for tissue engineering. Acta Biomaterialia, vol.10(2): 623–629. http://dx.doi.org/10.1016/j.actbio.2013.10.021
27. Whatley B R, Li X, Zhang N, et al., 2014, Magnetic-directed patterning of cell spheroids. Journal of Biomedical Materials Research A, vol.102(5): 1537–1547. http://dx.doi.org/10.1002/jbm.a.34797
28. Durmus N G, Tekin H C, Guven S, et al., 2015, Magnetic levitation of single cells. Proceedings of the National Academy of Science of the United States of America, vol.112(28): E3661–3668. http://dx.doi.org/10.1073/pnas.1509250112
29. Mirica K A, Ilievski F, Ellerbee A K, et al., 2011, Using magnetic levitation for three dimensional self-assembly. Advanced Materials, vol.23(36): 4134–4140. http://dx.doi.org/10.1002/adma.201101917
30. Tasoglu S, Yu C H, Liaudanskaya V, et al., 2015, Magnetic levitational assembly for living material fabrication. Advanced Healthcare Materials, vol.4(10): 1469–1476, 1422. http://dx.doi.org/10.1002/adhm.201500092
31. Kasyanov V, Brakke K, Vilbrandt T, et al., 2011, Toward organ printing: Design characteristics, virtual modelling and physical prototyping vascular segments of kidney arterial tree. Virtual and Physical Prototyping, vol.6(4): 197–213. http://dx.doi.org/10.1080/17452759.2011.631738
32. Xia L, Sakban R B, Qu Y, et al., 2012, Tethered spheroids as an in vitro hepatocyte model for drug safety screening. Biomaterials, vol.33(7): 2165–2176. http://dx.doi.org/10.1016/j.biomaterials.2011.12.006