Controlled preparation of droplets for cell encapsulation by air-focused microfluidic bioprinting
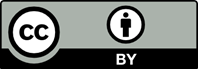
Microfluidics is a facile platform that manipulates fluids for the production of droplets, particles, and microcapsules. However, the application of microfluidics is limited to the manipulation of the droplet position and the presence of a continuous oil phase, which needs to be removed for biomedical applications. Here, we used air as the continuous phase and developed a facile method for droplet generation and patterning by air-focused microfluidic three-dimensional (3D) droplet printing (AFMDP). By tuning the viscous drag of a focused air flow, monodisperse droplets with tunable size were generated in a microfluidic device, and droplet patterns were designed by combining the control system of the 3D printer. When using droplets as templates, hydrogel particles were prepared by AFMDP and crosslinked in a CaCl2 bath. These hydrogel particles were proven to be good carriers for the cell culture, controlled release, and immune therapy using chimeric antigen receptor (CAR) T cells. Cell viability and activity results confirmed that encapsulation of CAR-T cells in hydrogel particles did not compromise their cell activity and functionality but facilitated their manipulation and cell culture. Therefore, the AFMDP system provided a versatile platform for the design of droplets, particles, and microcapsules for biomedical applications.
- Kong L, Chen R, Wang X, et al. Controlled co-precipitation of biocompatible colorant-loaded nanoparticles by microfluidics for natural color drinks. Lab Chip. 2019;19(12):2089-2095. doi: 10.1039/C9LC00240E
- Matsuura-Sawada Y, Maeki M, Nishioka T, et al. Microfluidic device-enabled mass production of lipid-based nanoparticles for applications in nanomedicine and cosmetics. ACS Appl Nano Mater. 2022;5(6):7867-7876. doi: 10.1021/acsanm.2c00886
- Tomeh MA, Zhao X. Recent advances in microfluidics for the preparation of drug and gene delivery systems. Mol Pharm. 2020;17(12):4421-4434. doi: 10.1021/acs.molpharmaceut.0c00913
- Sun J, Chen J, Liu K, Zeng H. Mechanically strong proteinaceous fibers: Engineered fabrication by microfluidics. Engineering. 2021;7(5):615-623. doi: 10.1016/j.eng.2021.02.005
- Chen L, Yang C, Xiao Y, et al. Millifluidics, microfluidics, and nanofluidics: Manipulating fluids at varying length scales. Mater Today Nano. 2021;16:100136. doi: 10.1016/j.mtnano.2021.100136
- Utada AS, Lorenceau E, Link DR, P D Kaplan, Stone HA, Weitz DA. Monodisperse double emulsions generated from a microcapillary device. Science. 2005;308(5721):537-541. doi: 10.1126/science.1109164
- Shang L, Cheng Yand ZY. Emerging droplet microfluidics. Chem Rev. 2017;117(12):7964-8040. doi: 10.1021/acs.chemrev.6b00848
- Xingzheng W, Zeyong S, Dong C. Preparation of droplet suspension by microfluidic technology. Modern Chemical Industry. 2020;40(9):70-74. doi: 10.16606/j.cnki.issn0253-4320.2020.09.015
- Gu Z, Xie M, Lv S, et al. Perfusable vessel-on-a-chip for antiangiogenic drug screening with coaxial bioprinting. Int J Bioprint. 2022;8(4):619. doi: 10.18063/ijb.v8i4.619
- Wu L, Guo Z, Liu W. Surface behaviors of droplet manipulation in microfluidics devices. Adv Colloid Interface Sci. 2022;308:102770. doi: 10.1016/j.cis.2022.102770
- Sun X, Wu Q, Li W, et al. Facile fabrication of drug-loaded PEGDA microcapsules for drug evaluation using droplet-based microchip. Chin Chem Lett. 2022;33(5):2697-2700. doi: 10.1016/j.cclet.2021.08.122
- Kim JH, Jeon TY, Choi TM, Shim TS, Kim S-H, Yang S-M. Droplet microfluidics for producing functional microparticles. Langmuir. 2014;30(6):1473-1488. doi: 10.1021/la403220p
- Liu W-Y, Ju X-J, Pu X-Q, et al. Functional capsules encapsulating molecular-recognizable nanogels for facile removal of organic micro-pollutants from water. Engineering. 2021;7(5):636-646. doi: 10.1016/j.eng.2021.02.007
- Shang L, Cheng Y, Wang J, et al. Double emulsions from a capillary array injection microfluidic device. Lab Chip. 2014;14(18):3489-3493. doi: 10.1039/C4LC00698D
- Zhai X, Pan M, Shi P, Zhao P, Chen D. One-step high-throughput controlled preparation of biocompatible water/ water microcapsules with triggered release. Chem J Chin Univ. 2022;43(12):10. doi: 10.7503/cjcu20220460
- Trantidou T, Elani Y, Parsons E, Ces O. Hydrophilic surface modification of PDMS for droplet microfluidics using a simple, quick, and robust method via PVA deposition. Microsyst Nanoeng. 2017;3(1):16091. doi: 10.1038/micronano.2016.91
- Chen D, Amstad E, Zhao C-X, et al. Biocompatible amphiphilic hydrogel–solid dimer particles as colloidal surfactants. ACS Nano. 2017;11(12):11978-11985. doi: 10.1021/acsnano.7b03110
- Choi C-H, Lee H, Weitz DA. Rapid patterning of PDMS microfluidic device wettability using syringe-vacuum-induced segmented flow in nonplanar geometry. ACS Appl Mater Interfaces. 2018;10(4):3170-3174. doi: 10.1021/acsami.7b17132
- Shah RK, Shum HC, Rowat AC, et al. Designer emulsions using microfluidics. Mater Today. 2008;11(4):18-27. doi: 10.1016/S1369-7021(08)70053-1
- Chen L, Xiao Y, Zhang Z, et al. Porous ultrathin-shell microcapsules designed by microfluidics for selective permeation and stimuli-triggered release. Front Chem Sci Eng. 2022;16(11):1643-1650. doi: 10.1007/s11705-022-2201-z
- Su Z, Hu W, Ye L, Gao Dan, Lin J-M. An integrated microfluidic chip-mass spectrometry system for rapid antimicrobial resistance analysis of bacteria producing β-lactamases. Chin Chem Lett. 2023;34(5):107790. doi: 10.1016/j.cclet.2022.107790
- Nam J, Lim H, Kim D, Jung H, Shin S. Continuous separation of microparticles in a microfluidic channel via the elasto-inertial effect of non-Newtonian fluid. Lab Chip. 2012;12(7):1347-1354. doi: 10.1039/C2LC21304D
- Sun Z, Yang C, Wang F, et al. Biocompatible and pH-responsive colloidal surfactants with tunable shape for controlled interfacial curvature. Angew Chem Int Ed. 2020;59(24):9365-9369. doi: 10.1002/anie.202001588
- Zhu J, Cai L-H. All-aqueous printing of viscoelastic droplets in yield-stress fluids. Acta Biomater. 2022;165(15):60-71. doi: 10.1016/j.actbio.2022.09.031
- Zhang M, Wangjin Y, Zhou H, et al. Fly ash and zero-valent iron-based in situ advanced anaerobic digestion with emphasis on the removal of antibiotics and antibiotic resistance genes from sewage sludge. Waste Dispos Sustain Energy. 2022;4:17-28. doi: 10.1007/s42768-021-00089-6
- Zhang H, Liu D, Shahbazi M-A, et al. Fabrication of a multifunctional nano-in-micro drug delivery platform by microfluidic templated encapsulation of porous silicon in polymer matrix. Adv Mater. 2014;26(26):4497-4503. doi: 10.1002/adma.201400953
- Liu Y, Yang G, Baby T, et al. Stable polymer nanoparticles with exceptionally high drug loading by sequential nanoprecipitation. Angew Chem Int Ed. 2020;59(12):4720-4728. doi: 10.1002/anie.201913539
- Chen Q, Utech S, Chen D, Prodanovic R, Lin J-M, Weitz DA. Controlled assembly of heterotypic cells in a core–shell scaffold: organ in a droplet. Lab Chip. 2016;16(8):1346-1349. doi: 10.1039/C6LC00231E
- Zhu J, He Y, Kong L, et al. Digital assembly of spherical viscoelastic bio-ink particles. Adv Funct Mater. 2022;32(6):2109004. doi: 10.1002/adfm.202109004
- Chae S, Ha D-H, Lee H. 3D bioprinting strategy for engineering vascularized tissue models. Int J Bioprint. 2023;9(5):15-33. doi: 10.18063/ijb.748
- Foresti D, Kroll KT, Amissah R, et al. Acoustophoretic printing. Sci Adv. 2018;4(8):eaat1659. doi: 10.1126/sciadv.aat1659
- Minemawari H, Yamada T, Matsui H, et al. Inkjet printing of single-crystal films. Nature. 2011;475(7356):364-367. doi: 10.1038/nature10313
- Reiser A, Lindén M, Rohner P, et al. Multi-metal electrohydrodynamic redox 3D printing at the submicron scale. Nat Commun. 2019;10(1):1853. doi: 10.1038/s41467-019-09827-1