Identification of potential drug targets for coronary atherosclerosis from genetic insights: A Mendelian randomization study
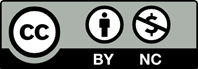
Coronary atherosclerosis (CA) is a chronic cardiovascular disease characterized by the accumulation of lipid plaques in coronary arteries, leading to vascular narrowing and impaired myocardial blood supply, which can result in severe complications, such as angina and myocardial infarction, and may even be life-threatening. Despite advancements in CA treatment, including medication and surgery, the complex mechanisms of plaque formation result in some patients responding poorly to existing therapies, and specific targets for precision treatment remain unclear. The present study utilized Mendelian randomization (MR) to investigate therapeutic targets for CA. Cis-expression quantitative trait loci (cis-eQTL, exposure data) were obtained from the eQTLGen consortium, which includes a sample of 31,684 individuals. Summary statistics for CA (outcome data) were obtained from the largest CA genome-wide association studies dataset, covering 456,348 individuals (including 16,041 cases and 440,307 controls), supplemented with data from the UK Biobank and FinnGen studies for external validation. Colocalization analysis was employed to examine whether CA risk and gene expression were driven by a shared single nucleotide polymorphism, thereby determining colocalized signals. Subsequently, drug prediction and molecular docking analysis were conducted to validate the druggability and pharmacological potential of the target genes, identifying promising therapeutic targets. The MR analysis identified nine drug targets, four of which were supported by colocalization, providing further evidence of their significance. Phenome-wide association studies analysis displayed no significant association between the DHX36 gene and other phenotypes. These genes potentially regulate CA by influencing specific metabolite levels. Molecular docking results indicated good binding affinity between multiple candidate drugs and proteins encoded by the target genes. Our study identified nine potential drug targets associated with CA, with four targets further supported for reliability. Given the roles of these genes in inflammation and metabolic regulation, drug development targeting these genes holds significant clinical potential. This study provides genetic evidence supporting the potential therapeutic benefits of targeting four druggable genes for CA treatment, which will aid in the development of CA drugs.
- Stone PH, Libby P, Boden WE. Fundamental pathobiology of coronary atherosclerosis and clinical implications for chronic ischemic heart disease management-the plaque hypothesis: A narrative review. JAMA Cardiol. 2023;8(2):192-201. doi: 10.1001/jamacardio.2022.3926
- Shao C, Wang J, Tian J, Tang YD. Coronary artery disease: From mechanism to clinical practice. Adv Exp Med Biol. 2020;1177:1-36. doi: 10.1007/978-981-15-2517-9_1
- Baaten C, Nagy M, Bergmeier W, Spronk HMH, van der Meijden PEJ. Platelet biology and function: Plaque erosion vs. rupture. Eur Heart J. 2024;45(1):18-31. doi: 10.1093/eurheartj/ehad720
- Milutinovic A, Suput D, Zorc-Pleskovic R. Pathogenesis of atherosclerosis in the tunica intima, media, and adventitia of coronary arteries: An updated review. Bosn J Basic Med Sci. 2020;20(1):21-30. doi: 10.17305/bjbms.2019.4320
- Duggan JP, Peters AS, Trachiotis GD, Antevil JL. Epidemiology of coronary artery disease. Surg Clin North Am. 2022;102(3):499-516. doi: 10.1016/j.suc.2022.01.007
- Gawaz M, Geisler T, Borst O. Current concepts and novel targets for antiplatelet therapy. Nat Rev Cardiol. 2023;20(9):583-599. doi: 10.1038/s41569-023-00854-6
- Eghtedari B, Roy SK, Budoff MJ. Anti-inflammatory therapeutics and coronary artery disease. Cardiol Rev. 2023;31(2):80-86.doi: 10.1097/CRD.0000000000000428
- Oikonomou E, Siasos G, Tsigkou V, et al. Coronary artery disease and endothelial dysfunction: Novel diagnostic and therapeutic approaches. Curr Med Chem. 2020;27(7):1052-1080. doi: 10.2174/0929867326666190830103219
- Serruys PW, Ono M, Garg S, et al. Percutaneous coronary revascularization: JACC historical breakthroughs in perspective. J Am Coll Cardiol. 2021;78(4):384-407. doi: 10.1016/j.jacc.2021.05.024
- Grover MP, Ballouz S, Mohanasundaram KA, et al. Novel therapeutics for coronary artery disease from genome-wide association study data. BMC Med Genomics. 2015;8 Suppl 2(Suppl 2):S1. doi: 10.1186/1755-8794-8-S2-S1
- Hughes MF, Lenighan YM, Godson C, Roche HM. Exploring coronary artery disease GWAs targets with functional links to immunometabolism. Front Cardiovasc Med. 2018;5:148. doi: 10.3389/fcvm.2018.00148
- Storm CS, Kia DA, Almramhi MM, et al. Finding genetically-supported drug targets for Parkinson’s disease using Mendelian randomization of the druggable genome. Nat Commun. 2021;12(1):7342. doi: 10.1038/s41467-021-26280-1
- Finan C, Gaulton A, Kruger FA, et al. The druggable genome and support for target identification and validation in drug development. Sci Transl Med. 2017;9(383):eaag1166. doi: 10.1126/scitranslmed.aag1166
- Hemani G, Zheng J, Elsworth B, et al. The MR-Base platform supports systematic causal inference across the human phenome. Elife. 2018;7:e34408. doi: 10.7554/eLife.34408
- Greco MF, Minelli C, Sheehan NA, Thompson JR. Detecting pleiotropy in Mendelian randomisation studies with summary data and a continuous outcome. Stat Med. 2015;34(21):2926-2940. doi: 10.1002/sim.6522
- Giambartolomei C, Vukcevic D, Schadt EE, et al. Bayesian test for colocalisation between pairs of genetic association studies using summary statistics. PLoS Genet. 2014;10(5):e1004383. doi: 10.1371/journal.pgen.1004383
- Wang Q, Dhindsa RS, Carss K, et al. Rare variant contribution to human disease in 281,104 UK Biobank exomes. Nature. 2021;597(7877):527-532. doi: 10.1038/s41586-021-03855-y
- Yu G, Wang LG, Han Y, He QY. clusterProfiler: An R package for comparing biological themes among gene clusters. OMICS. 2012;16(5):284-287. doi: 10.1089/omi.2011.0118
- Otasek D, Morris JH, Boucas J, Pico AR, Demchak B. Cytoscape automation: Empowering workflow-based network analysis. Genome Biol. 2019;20(1):185. doi: 10.1186/s13059-019-1758-4
- Yoo M, Shin J, Kim J, et al. DSigDB: Drug signatures database for gene set analysis. Bioinformatics. 2015;31(18):3069-3071. doi: 10.1093/bioinformatics/btv313
- Shaffer JM, Smithgall TE. Promoter methylation blocks FES protein-tyrosine kinase gene expression in colorectal cancer. Genes Chromosomes Cancer. 2009;48(3):272-284. doi: 10.1002/gcc.20638
- Li J, Smithgall TE. Fibroblast transformation by Fps/Fes tyrosine kinases requires Ras, Rac, and Cdc42 and induces extracellular signal-regulated and c-Jun N-terminal kinase activation. J Biol Chem. 1998;273(22):13828-13834. doi: 10.1074/jbc.273.22.13828
- Laurent CE, Smithgall TE. The c-Fes tyrosine kinase cooperates with the breakpoint cluster region protein (Bcr) to induce neurite extension in a Rac- and Cdc42-dependent manner. Exp Cell Res. 2004;299(1):188-198. doi: 10.1016/j.yexcr.2004.05.010
- Chen Y, Yang Z, Meng M, et al. Cullin mediates degradation of RhoA through evolutionarily conserved BTB adaptors to control actin cytoskeleton structure and cell movement. Mol Cell. 2009;35(6):841-855. doi: 10.1016/j.molcel.2009.09.004
- Karamanavi E, McVey DG, van der Laan SW, et al. The FES Gene at the 15q26 coronary-artery-disease locus inhibits atherosclerosis. Circ Res. 2022;131(12):1004-1017. doi: 10.1161/CIRCRESAHA.122.321146
- Antcliff A, McCullough LD, Tsvetkov AS. G-Quadruplexes and the DNA/RNA helicase DHX36 in health, disease, and aging. Aging (Albany NY). 2021;13(23):25578-25587. doi: 10.18632/aging.203738
- Zeng Y, Qin T, Flamini V, et al. Identification of DHX36 as a tumour suppressor through modulating the activities of the stress-associated proteins and cyclin-dependent kinases in breast cancer. Am J Cancer Res. 2020;10(12):4211-4233.
- Lai JC, Ponti S, Pan D, et al. The DEAH-box helicase RHAU is an essential gene and critical for mouse hematopoiesis. Blood. 2012;119(18):4291-4300. doi: 10.1182/blood-2011-08-362954
- Nikpay M, Soubeyrand S, Tahmasbi R, McPherson R. Multiomics screening identifies molecular biomarkers causally associated with the risk of coronary artery disease. Circ Genom Precis Med. 2020;13(6):e002876. doi: 10.1161/CIRCGEN.119.002876
- Li R, Zhang H, Tang F, et al. Coronary artery disease risk factors affected by RNA modification-related genetic variants. Front Cardiovasc Med. 2022;9:985121. doi: 10.3389/fcvm.2022.985121
- Wallach D. The cybernetics of TNF: Old views and newer ones. Semin Cell Dev Biol. 2016;50:105-114. doi: 10.1016/j.semcdb.2015.10.014
- Andersson J, Sundstrom J, Kurland L, et al. The carotid artery plaque size and echogenicity are related to different cardiovascular risk factors in the elderly: The prospective investigation of the vasculature in Uppsala seniors (PIVUS) study. Lipids. 2009;44(5):397-403. doi: 10.1007/s11745-009-3281-y
- Zhang H, Jiang M, Hou H, Li Q. Efficacy of simvastatin on carotid atherosclerotic plaque and its effects on serum inflammatory factors and cardiocerebrovascular events in elderly patients. Exp Ther Med. 2021;22(2):819. doi: 10.3892/etm.2021.10251
- Mourouzis K, Oikonomou E, Siasos G, et al. Pro-inflammatory cytokines in acute coronary syndromes. Curr Pharm Des. 2020;26(36):4624-4647. doi: 10.2174/1381612826666200413082353
- van Loo G, Bertrand MJM. Death by TNF: A road to inflammation. Nat Rev Immunol. 2023;23(5):289-303. doi: 10.1038/s41577-022-00792-3
- Armstrong AW, Read C. Pathophysiology, clinical presentation, and treatment of psoriasis: A review. JAMA. 2020;323(19):1945-1960. doi: 10.1001/jama.2020.4006
- Wride AM, Chen GF, Spaulding SL, Tkachenko E, Cohen JM. Biologics for psoriasis. Dermatol Clin. 2024;42(3):339-355. doi: 10.1016/j.det.2024.02.001
- Efferth T, Oesch F. The immunosuppressive activity of artemisinin-type drugs towards inflammatory and autoimmune diseases. Med Res Rev. 2021;41(6):3023-3061. doi: 10.1002/med.21842
- Shi Z, Chen Y, Lu C, et al. Resolving neuroinflammation, the therapeutic potential of the anti-malaria drug family of artemisinin. Pharmacol Res. 2018;136:172-180. doi: 10.1016/j.phrs.2018.09.002
- Huang Y, Chen L, Li L, et al. Downregulation of adipose LPL by PAR2 contributes to the development of hypertriglyceridemia. JCI Insight. 2024;9(13):e173240. doi: 10.1172/jci.insight.173240
- Xie L, Li YM. Lipoprotein lipase (LPL) polymorphism and the risk of coronary artery disease: A meta-analysis. Int J Environ Res Public Health. 2017;14(1):84. doi: 10.3390/ijerph14010084