Validation of Design Methodology for Rainwater Harvesting for Tropical Climates
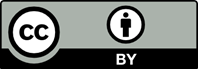
Promotion of sustainable development in the building sector with reduced embodied energy and life cycle costs has become a primary need for facing the emerging threats of global warming and depleting energy sources. In this context, the minimization of the dependence on reticulated water supply is of crucial importance due to the high level of operational energy and the cost associated with the reticulated water supply. For this purpose, rainwater harvesting (RWH) can offer an ideal solution where the harvested water can be easily used for flushing of toilets and gardening. Though design curves and parameters are available for design of the rainwater tanks for a significant water saving efficiency (WSE) in temperate climate regions, the validity and the applicability of these curves and parameters for tropical climate regions such as Sri Lanka, where rainfall patterns vary significantly across geographical and climatic boundaries, is still limited. It is also important to utilize a practical solution to develop multiple data, using short-term rainfall data, in order to compensate for the lack of available data for many geographical regions. In this research, a practical methodology was employed using short-term rainfall data and an experimental set up to validate design curves for WSE, obtained in temperate climate regions, to predict the WSE for tropical climate regions.
Basinger, M., Montalto, F. and U. Lall (2010). A rainwater harvesting system reliability model based on nonparametric stochastic rainfall generator. Journal of Hydrology, 392(3-4): 105-118.
Boers, Th.M. and J. Ben-Asher (1982). A Review of Rainwater Harvesting. Agricultural Water Management,
5(2): 145-158.
Dixon, A., Butler, D. and A. Fewkes (1999). Computer simulation of domestic water reuse systems: Investigating grey water and rainwater in combination. Water Science Technology, 38(4): 25-32.
Fewkes, A. (1999a). The use of rainwater for WC flushing: The field-testing of a collection system. Building and Environment, 34: 765-772.
Fewkes, A. (1999b). Modelling the performance of rainwater collection systems: Towards a generalized approach. Urban Water, 1: 323-333.
Fewkes, A. and D. Butler (2000). Simulating the performance of rainwater collection systems using behavioural models. Building Services, Engineering Research and Technology,21(2): 99-106.
Handia, L., Madalitso, T. and C. Mwiindwa (2003). Potential of rainwater harvesting in urban Zambia. Physics and Chemistry of Earth, 28(20-27): 893-896.
Hermann, T. and U. Schmida (1999). Rainwater utilization in Germany: Efficiency, dimensioning, hydraulic and environmental aspects. Urban Water, 1: 307-316.
Kahinda, J.M., Taigbenu, A.E. and J.R. Boroto (2007). Domestic rainwater harvesting to improve water supply in rural South Africa. Physics and Chemistry of the Earth, Parts A/B/C, 32(15-18): 1050-1057.
Luxmore, D.A., Jayasinghe, M.T.R. and M. Mahendran (2005). Mitigating temperature increase in high lot density sub tropical residential developments. Energy and Buildings, 37: 1212-1224.
Villareal, E.L. and A. Dixon (2005). Analysis of a rainwater collection system for domestic water supply in Ringdansen, Norrkoping, Sweden. Building and Environment, 40(9): 1174-1184.
Zobrist, J., Muller, S.R., Amman, A., Bucheli, T.A., Mottier, V., Ochs, M., Shoenenberger, R., Engster, J. and M. Boller
(2000). Quality of roof run-off for groundwater infiltration. Water Resources, 34(5): 1455-1462.